Biochemical and physiological tolerance evaluation of the oil-yielding paradise tree ( Simarouba amara Aubl.) under salt stress
Abstract
Simarouba amara Aubl., a native of tropical regions of South and Central America was introduced in India for wasteland reclamation purposes. Present study was conducted to evaluate its ability to grow under salt stress conditions. S. amara seedlings were exposed to different concentrations of sodium chloride (50 mM, 100 mM, 150 mM, and 200 mM). Morphologically, at higher salt concentrations (150 and 200 mM NaCl), S. amara seedlings showed symptoms of leaf chlorosis, wilting, and drooping. A significant reduction in leaf relative water content, photosynthetic pigments and increase in lipid peroxidation was observed under high salt stress conditions. Under salt stress, levels of both enzymatic as well as non-enzymatic antioxidants increased majorly. At 200 mM NaCl, proline and cysteine content increased by 116% and 166% respectively. Among antioxidant enzymes, maximum increase in activity was observed in glutathione reductase (320%) followed by superoxide dismutase (129%), ascorbate peroxidase (80%), guaiacol peroxidase (80%) and catalase (59%) at 200 mM NaCl. A significant increase in the activities of both enzymatic and non-enzymatic antioxidants reveals that S. amara has better defensive mechanism against salt stress, but up to 100 mM concentration. At 150 and 200 mM NaCl, salt toxicity hampered growth of the plants.
Keywords
Simarouba amara, antioxidants, lipid peroxidation, Salt stress
Introduction
The world population is expected to be cross 9 billion by 2050 and with such fast growing numbers, there is an extreme stress on agriculture to satisfy the demands of food, fodder, fiber, etc. (Ashraf, 2020). Agriculture is also getting affected due to various factors such as huge reduction in cultivable lands, urbanization, unusual weather patterns, etc. Among different environmental stress that have negative impact on agriculture, enhancement of salinity in cultivable lands (soil salinity) is the most prevalent one that hampers the crop productivity majorly. Soil salinity takes place due to various natural processes like weathering, sea water deposition, etc. or due to different human activities (irrigation with brackish ground water, improper drainage system, etc.) (Evelin, 2019). Such salt affected lands are not fertile and are not suitable for crop production/ agriculture. Due to salt stress, different phases of plant life are affected such as seed germination, vegetative growth and reproducibility, consequently leading to reduced growth and development in plants. Toxic effects of salt stress on plants include osmotic stress and oxidative stress due to reactive oxygen species (ROS) production which further lead to osmotic and ionic imbalance, damage to functional, structural proteins, DNA, and cell membrane, reduced photosynthesis, etc. (Shrivastava & Kumar, 2015); (Tanveer & Yousaf, 2020). Ion toxicity (due to high Na+ concentration) in plants affects various physiological activities which may lead to chlorosis and necrosis (Acosta-Motos, 2017). To mitigate the toxic effects of soil salinity, plants also have defensive mechanism which includes activation of enzymatic and non-enzymatic antioxidants, ion homeostasis, formation of compatible osmolytes, polyamines, nitric oxides, etc. Adaptive mechanism under salt stress conditions also include morphological changes. Thus, it is of high importance to explore some novel plants which have better defensive system and can tolerate salt stress conditions and can be grown/cultivated under high soil salinity conditions. Effect of salt stress has been recently investigated in various plants such as Vigna unguiculata (El-Taher et al., 2021), Lactuca sativa (Bres et al., 2022), Phaseolus vulgaris (Al-huraby and Bafeel, 2022), Reaumuria soongorica (Yan et al., 2022), Capsicum annuum (Sarwar et al., 2022), etc. In plants, salt tolerant mechanism includes avoidance (salt exclusion, salt dilution, etc.) and tolerance (osmotic adjustment, hormone synthesis, etc.). Various heavy salt tolerant tree species are Populus euphratica, Tamarix chinensis, Cocos nucifera, Avicennia marina, Pyrus betulaefolia, Rhus Typhina, etc. Moderate salt tolerant tree species include Hippophae rhamnoides, Eucalyptus robusta, Pistacia chinensis, Gleditsia sinensis, Quercus rubra, etc. Light salt tolerant tree species are Jatropha curcas, Ginkgo biloba, Pistacia chinensis, etc. (Zhang, 2021).
Simarouba amara Aubl., commonly known as paradise tree, belongs to Simaroubaceae family and is a native of tropical regions of South and Central America. It is a polygamodioecious, medium sized, evergreen, and multipurpose oil seed tree that is considered to adapt and grow well on wastelands, therefore useful for wasteland reclamation (Joshi & Joshi, 2008). The tree has well-developed roots, prevents soil erosion and improves soil fertility. In addition, this plant was introduced in India from El Salvador by National Bureau of Plant Genetic Resources (NBPGR) especially for soil conservation and wasteland reclamation purposes. However its ability to grow under salt stress (soil salinity) has not been studied yet. In S. amara, effect of water stress and its amelioration through foliar application of salicylic acid have been investigated by (Awate & Gaikwad, 2014).
Thus, the aim of the present study was to determine the effect of salinity on S. amara plants. As seedlings growth stages of most of the crop plants are the most sensitive stages different stress (Cuartero, 2006), two-month-old seedlings were preferred for the stress study.
Materials and methods
Plant material and stress treatments
Seeds of S. amara were procured from University of Agriculture Sciences, Bangalore, India. Seeds were surface sterilized with 5% sodium hypochlorite solution for 20 minutes and then washed thoroughly with distilled water. Seeds were sown in clay pots containing autoclaved soil and were allowed to germinate under natural conditions of light, temperature and humidity. After 15-25 days of sowing, S. amara seeds started germination. Two-month-old uniform seedlings were transferred to other clay pots containing 4 kg of autoclaved sandy loam soil exposed to different concentrations of NaCl (0 mM, 50 mM, 100 mM, 150 mM, 200 mM). In each pot, three seedlings were planted and pots were placed in green house with 25±2ºC and 55% relative humidity. Each of these treatments had three replicates and pots without the addition of NaCl served as control. The plants were harvested after 21 days of stress treatment Figure 1 and kept in -80ºC for further experiments. All the fine chemicals used in the study were procured from Merck, Life Science, India.
Phenotypic assay
Morphological traits of all the S. amara plants after 21 days of stress treatment were analyzed. Leaf relative water content was determined following the method of (Turner & Kramer, 1980) and calculated by the equation: LRWC(%)=[(Dry weight of sample-Fresh weight of sample)/(Turbid weight of sample-Fresh weight of sample)] x 100.
MDA estimation
MDA concentration was determined following the method of (Heath & Packer, 1968). For each reaction, 250 mg of leaf material was homogenized in 5 mL of 1% trichloroacetic acid (TCA) and centrifuged at 8,500 x g for 10 min at 4ºC, after which 1 mL supernatant was collected and was mixed with 4 mL of TCA/TBA reagent (0.5% TBA in 20% TCA) and then heated at 95ºC for 30 min. After incubation, samples were centrifuged at 10,000 x g for 10 min at 4ºC and specific and non- specific absorbance was measured spectrophotometrically at 535 and 600 nm, respectively.
Photosynthetic pigments
Leaf discs (100 mg) were soaked in 80% (v/v) acetone solution and kept in dark overnight. The absorbance of the acetone extracts was measured at 663, 645 and 470 nm. The concentrations of chlorophyll a and chlorophyll b were calculated using Arnon’s equations (Arnon, 1949) and carotenoids content was measured following formula given byLichtenthaler and Wellburn (1983).
Determination of sodium ions
Quantification of sodium ions in the aqueous extract of S. amara leaves was done by following protocol given byWeimberg (1987) with a flame photometer.
Non-enzymatic antioxidants
Proline estimation
Proline content was measured by following protocol given byBates (1973). Proline was extracted from 200 mg of leaves with 5 mL of 3% sulfosalicylic acid. The homogenate was centrifuged at 15,000 x g for 10 min at 4ºC and 2 mL of supernatant obtained was reacted with 2 mL of glacial acetic acid and 2 mL of acid ninhydrin. The reaction mixture was heated at 100ºC for 1 hr followed by cooling in ice. Then, 4mL of toluene was added with vortexing for diffusion of chromophore from aqueous to non-aqueous phase. Absorbance of aqueous phase was measured at 520 nm and proline concentration was determined from proline standard curve.
Cysteine estimation
Gaitonde method (1967) was followed for determination of cysteine content which was extracted from 250 mg of leaves with 5 mL of 5% chilled perchloric acid (v/v). The homogenate was centrifuged at 10,000 x g for 10 min at 4ºC. Supernatant obtained (0.5 mL) was added to 0.5 mL of acid ninhydrin reagent II and 0.5 ml of glacial acetic acid. The reaction mixture was heated at 100ºC for 15 min and pink color was developed. The absorbance was measured at 560 nm.
Antioxidant enzyme assays
S. amara leaves (1 g) were ground to powder in liquid nitrogen using pre-chilled mortar and pestle. The powder was homogenized with 4 mL of chilled 0.2 M phosphate buffer (pH 7.8) containing 0.1 mM EDTA. The homogenate was centrifuged at 13,000 x g at 4ºC for 20 min. The supernatant was used for all the enzyme activity assays. Total soluble protein of the extract was measured following Bradford assay (Bradford, 1976) employing bovine serum albumin (BSA) as the standard. All the assays were performed thrice.
SOD (EC 1 15 1 1 assay
Superoxide dismutase activity was determined employing modified NBT method (Beyer & Fridovich, 1987) .The reaction mixture with a total volume of 3 mL contained 60 mM phosphate buffer (pH 7.5), 2 µM riboflavin, 0.1 mM Na2EDTA, 13 mM L-methionine, 70 µM NBT and 50 µL of enzyme extract. Samples were incubated for 15 min under two 15 W fluorescent tubes. Absorbance was recorded spectrophotometrically for 3 min at 560 nm.
CAT (EC 1 11 1 6 assay
Catalase activity was measured by the procedure described byAebi (1984). CAT activity was initiated by adding 50 µL enzyme extract into the reaction mixture containing 90 mM phosphate buffer (pH 7.0) and H2O2 (18 mM). Decomposition of H2O2 was measured spectrophotometrically at 240 nm for 100 s at 25ºC.
GPX (EC 1 11 1 7 assay
Guaiacol peroxidase assay was conducted following the method of Thimmaiah (1999). The reaction mixture (3 mL) contained 65 mM phosphate buffer (pH 6.0), 2.25 mM guaiacol, 11 mM H2O2 and 50 µL enzyme extract. The increase in absorbance was monitored spectrophotometrically at 470 nm for 300 s at 25ºC.
APX (EC 1 11 1 11 assay
Ascorbate peroxidase assay was conducted employing modified method of Nakano and Asada (1981) . The reaction mixture contained 80 mM phosphate buffer (pH 7.0), 0.5 mM ascorbic acid, 0.6 mM H2O2, 0.1 mM EDTA, and 0.2 mL enzyme extract. Absorbance changes were recorded spectrophotometrically for 500 s at 290 nm.
GR (EC 1 6 4 2 assay
Glutathione reductase activity was measured following the protocol ofSchaedle and Bassham (1977). Enzymatic reaction was initiated by adding 50 µL enzyme extract to the reaction mixture containing 60 mM phosphate buffer (pH 7.5), 10 mM NADPH, 10 mM GSSG and 6.25 mM MgCl2. Changes in absorbance were measured spectrophotometrically at 340 nm for 15 min.
Statistical analysis
All data in the present study was represented as mean ± standard deviation of the mean (SD) and was analyzed employing one-way ANOVA through SPSS version 22.0 software. The differences between treatments were detected using Duncan’s multiple range tests (P<0.05).
Results
Morphology analysis and leaf relative water content (LRWC determination
Under salt stress conditions, best plant growth was observed at 50 mM NaCl followed by 100 mM NaCl concentration. At higher salt concentrations (150 and 200 mM NaCl), S. amara seedlings showed symptoms of leaf chlorosis, wilting, and drooping. Leaf relative water content (LRWC) of control seedlings was found to be 69.74% which further reduced when exposed to different concentrations of NaCl. NaCl treated seedlings exhibited 59.55%, 57.84%, 54.62% and 48.37% LRWC at 50 mM, 100 mM, 150 mM and 200 mM concentrations respectively Figure 2. Decrease in LRWC at maximum NaCl concentration (200 mM) was 30% Table 1.
MDA estimation
S. amara seedlings showed increase in MDA content when exposed to different NaCl concentrations. Control seedlings exhibited 13.12 µmol g-1 FW MDA content and with increase in stress concentrations, MDA content enhanced significantly (Figure 2D-F). MDA content was found to be 14.19 µmol g-1 FW, 21.07 µmol g-1 FW, 23.44 µmol g-1 FW and 24.73 µmol g-1 FW at 50 mM, 100 mM, 150 mM and 200 mM NaCl concentrations respectively (Figure 2D). Percentage increase in MDA content at maximum NaCl concentration (200 mM) was 88% (Table 1).
Photosynthetic pigments
NaCl stress resulted in decrease in photosynthetic pigments (chlorophyll a, chlorophyll b and carotenoids) in S. amara seedlings (Figure 2G-J). Control seedlings exhibited 1.43 mg g-1 FW, 1.003 mg g-1 FW and 10.44 mg g-1 FW chlorophyll a, chlorophyll b and carotenoids contents respectively and with increase in stress concentrations, photosynthetic pigments reduced significantly. S. amara seedlings treated with different NaCl concentrations (50 mM, 100 mM, 150 mM and 200 mM), exhibited 0.67 mg g-1 FW, 1.03 mg g-1 FW, 0.88 mg g-1 FW and 0.77 mg g-1 FW chlorophyll a content respectively. At same NaCl range, chlorophyll b content was found to be 0.63 mg g-1 FW, 0.47 mg g-1 FW, 0.11 mg g-1 FW and 0.07 mg g-1 FW respectively. Carotenoids content was 9.32 mg g-1 FW, 9.87 mg g-1 FW, 5.27 mg g-1 FW and 5.06 mg g-1 FW respectively at similar NaCl range (Figure 2G). Decrease in chlorophyll a, chlorophyll b and carotenoids content at maximum NaCl concentration (200 mM) was 46%, 93% and 51% respectively (Table 1).
Determination of sodium ions (Na+)
Content of sodium ions (Na+) in the leaf samples of S. amara seedlings exposed to 0 mM, 50 mM, 100 mM, 150 mM and 200 mM NaCl concentrations was found to be 305 μmol g-1 DW, 525 μmol g-1 DW, 764 μmol g-1 DW, 1156 μmol g-1 DW, 1450 μmol g-1 DW, respectively.
Non-enzymatic antioxidants
Proline estimation
NaCl stress resulted in increase in proline content. Control seedlings exhibited 26.19 µmol g-1 FW proline content. Proline concentration significantly increased with increase in different stress concentrations (Figure 3A-C). S. amara seedlings exposed to increasing NaCl concentrations (50 mM, 100 mM, 150 mM and 200 mM) revealed proline content of 27.63 µmol g-1 FW, 35.5 µmol g-1 FW, 37.44 µmol g-1 FW and 56.74 µmol g-1 FW respectively (Figure 3A). Increase in proline content at 200 mM NaCl concentration was found to be 116% (Table 1).
Control |
NaCl |
||
---|---|---|---|
|
|
200 mM |
% ↑ or ↓ |
LRWC (%) |
69.74 |
48.37 |
↓ 30.64 |
MDA content (µmol g-1 FW) |
13.12 |
24.73 |
↑ 88.49 |
Chl. a content (mg g-1 FW) |
1.43 |
0.77 |
↓ 46.15 |
Chl. b content (mg g-1 FW) |
1.003 |
0.07 |
↓ 93.02 |
Carotenoids content (mg g-1 FW) |
10.44 |
5.06 |
↓ 51.53 |
SOD activity (Unit mgprotein -1) |
6.25 |
14.35 |
↑ 129.60 |
CAT activity (mmolH2O2min-1 mgprot -1) |
0.62 |
0.99 |
↑ 59.68 |
APX activity (mmolVit.Cmin-1 mgprot -1) |
1.57 |
2.84 |
↑ 80.89 |
GPX activity (mmoltetraguaiacol min-1 mgprot -1) |
0.05 |
0.09 |
↑ 80.00 |
GR activity (mmolNADPH min-1 mgprot -1) |
0.05 |
0.21 |
↑ 320.00 |
Proline content (µmol g-1 FW) |
26.19 |
56.74 |
↑ 116.65 |
Cysteine content (µmol g-1 FW) |
20.17 |
53.84 |
↑ 166.93 |
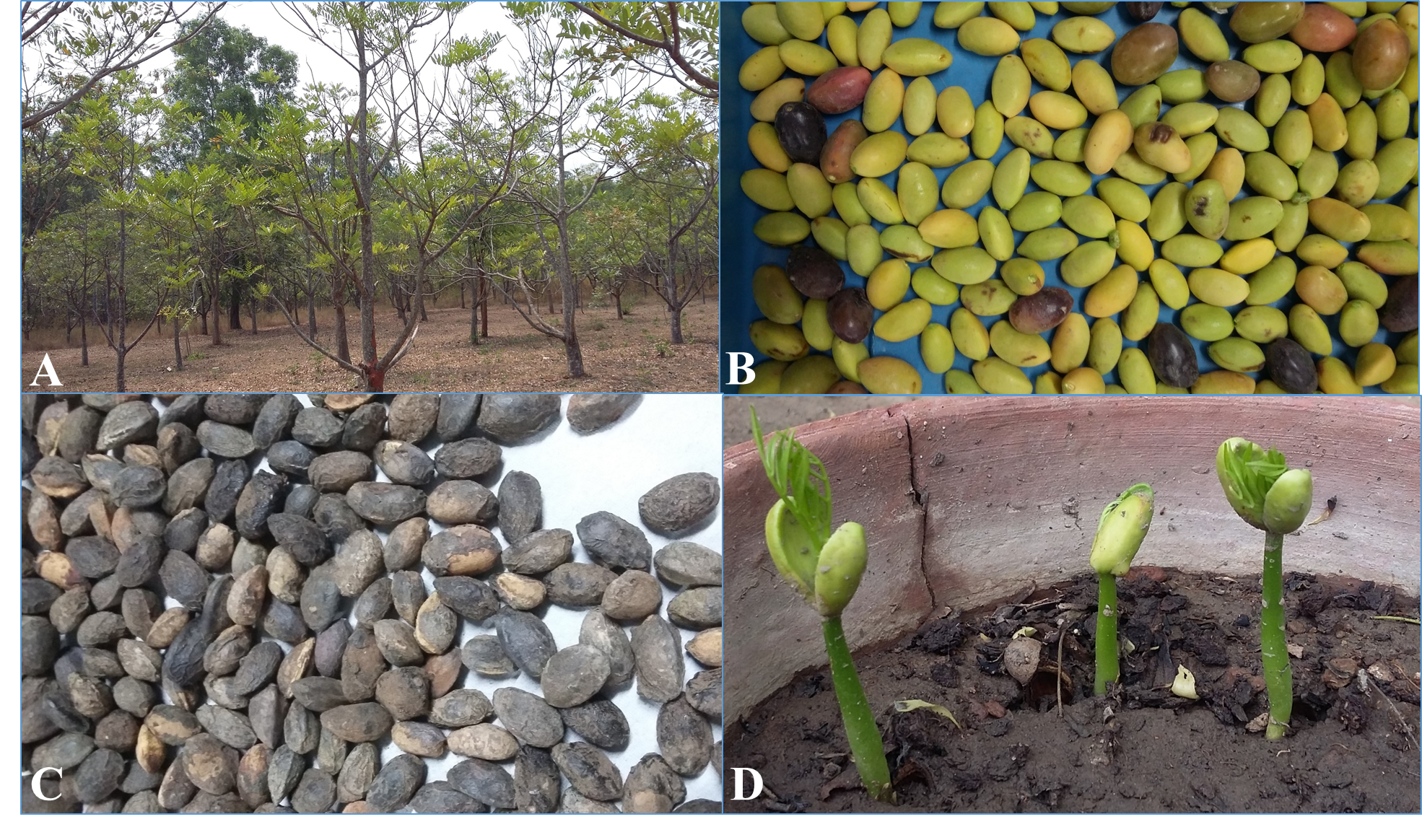
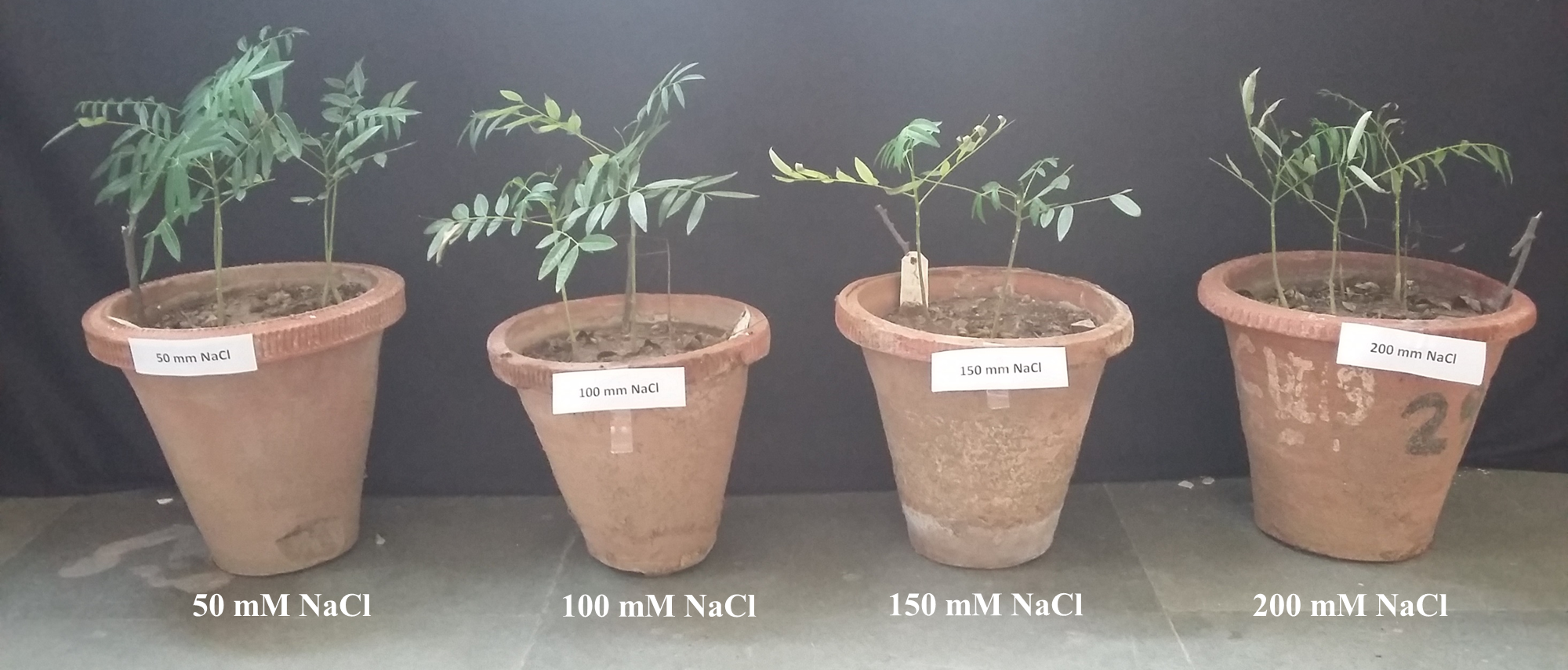
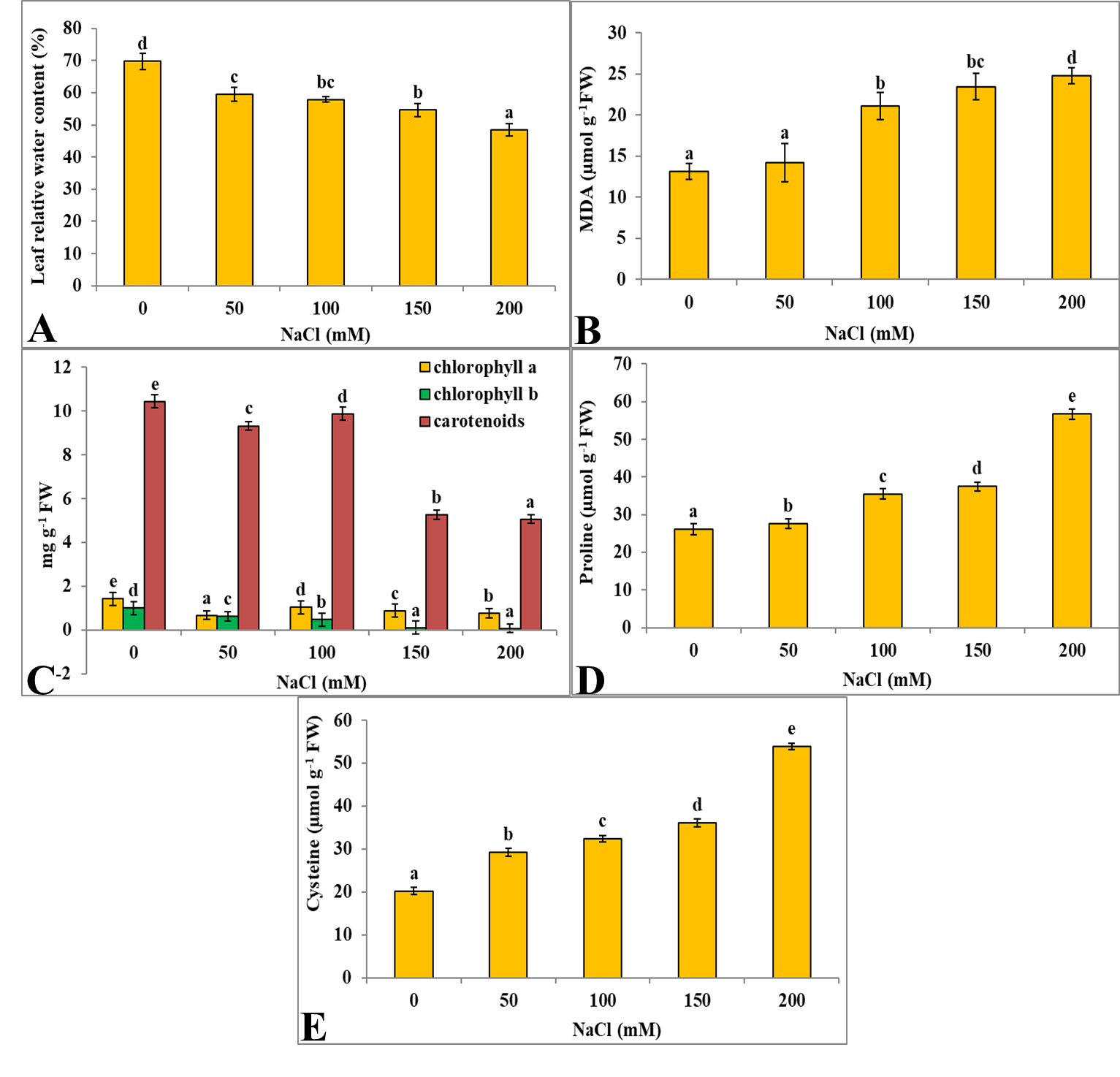
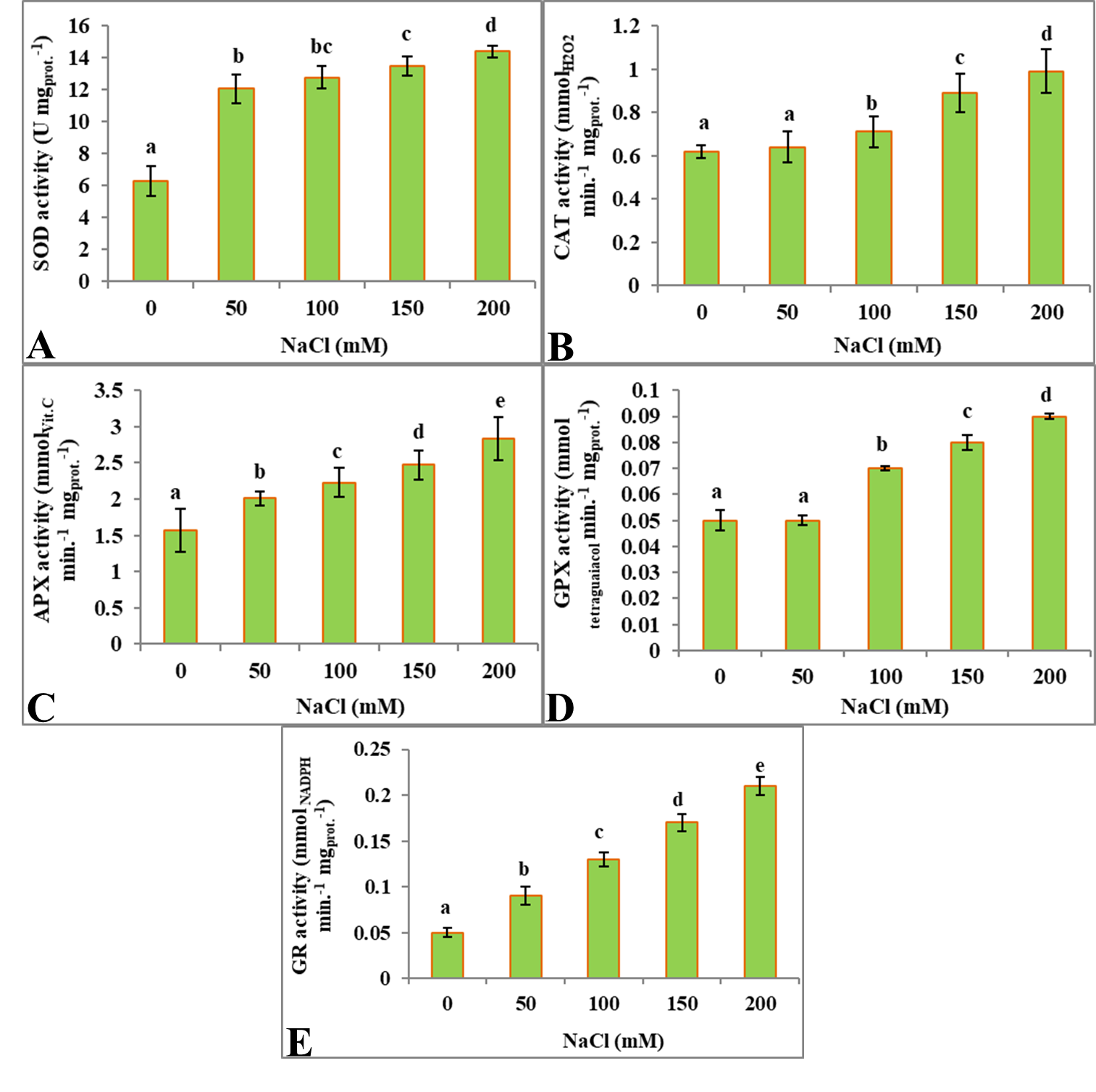
Cysteine estimation
S. amara seedlings exposed to NaCl stress resulted in increase in cysteine concentration. Control seedlings exhibited 20.17 µmol g-1 FW cysteine content and with increase in stress concentrations, cysteine content enhanced significantly (Figure 3D-F). Cysteine content was 29.28 µmol g-1 FW, 32.41 µmol g-1 FW, 36.07 µmol g-1 FW and 53.84 µmol g-1 FW in NaCl treated seedlings at 50 mM, 100 mM, 150 mM and 200 mM concentrations respectively (Figure 3D). Percentage increase in cysteine content at maximum NaCl concentration (200 mM) was 166% (Table 1).
Antioxidant enzyme assays
SOD assay:
In S. amara seedlings, NaCl stress resulted in increase in SOD activity. Control seedlings exhibited 6.25 Unit mgprotein -1 SOD activity and with increase in different stresses concentrations, SOD activity increased significantly in each case (Figure 4A,F, K,P). SOD activity was 12.03 Unit mgprotein -1, 12.74 Unit mgprotein -1, 13.47 Unit mgprotein -1 and 14.35 Unit mgprotein -1 in S. amara seedlings exposed to 50 mM, 100 mM, 150 mM and 200 mM NaCl concentrations respectively (Figure 4A). SOD activity increased 129% at maximum NaCl concentration (200 mM) (Table 1).
CAT assay:
A significant increase in CAT activity was revealed, when S. amara seedlings were exposed to different concentrations of NaCl. CAT activity of control seedlings was found to be 0.62 mmolH2O2min-1 mgprot -1 and it increased significantly due to each stress (Figure 4B,G, L,Q). NaCl treated seedlings resulted in CAT activity of 0.64 mmolH2O2min-1 mgprot -1, 0.71 mmolH2O2min-1 mgprot -1, 0.89 mmolH2O2min-1 mgprot -1and 0.99 mmolH2O2min-1 mgprot -1in at 50 mM, 100 mM, 150 mM and 200 mM concentrations respectively (Figure 4B). CAT activity increased by 59% at 200 mM NaCl concentration (Table 1).
APX assay:
APX activity increased significantly in S. amara seedlings treated with different concentrations of NaCl. Control seedlings exhibited 1.57 mmolVit.Cmin-1 mgprot -1 of APX activity which increased significantly in each case (Figure 4C,H, M,R). APX activity was 2.01 mmolVit.Cmin-1 mgprot -1, 2.23 mmolVit.Cmin-1 mgprot -1, 2.47 mmolVit.Cmin-1 mgprot -1 and 2.84 mmolVit.Cmin-1 mgprot -1 in NaCl treated seedlings at 50 mM, 100 mM, 150 mM and 200 mM concentrations respectively (Figure 4C). APX activity increased by 80% at 200 mM NaCl concentration (Table 1).
GPX assay:
GPX activity of control seedlings was found to be 0.05 mmoltetraguaiacol min-1 mgprot -1. Compared to control seedlings, GPX activity was found higher in S. amara seedlings exposed to different NaCl concentrations. GPX activity increased significantly with increase in concentrations of each stress treatment (Figure 4D,I, N,S). In NaCl treated seedlings, GPX activity was 0.05 mmoltetraguaiacol min-1 mgprot -1, 0.07 mmoltetraguaiacol min-1 mgprot -1, 0.08 mmoltetraguaiacol min-1 mgprot -1and 0.09 mmoltetraguaiacol min-1 mgprot -1at 50 mM, 100 mM, 150 mM and 200 mM of NaCl concentrations respectively (Figure 4D). GPX activity increased by 80% at maximum NaCl concentration (200 mM) (Table 1).
GR assay:
GR activity significantly increased in S. amara seedlings exposed to different NaCl concentrations. Control seedlings exhibited 0.05 mmolNADPH min-1 mgprot -1 of GR activity, which significantly increased with increase in stress concentrations in each case (Figure 4E, J, O, T). In NaCl treated S. amara seedlings, GR activity was 0.09 mmolNADPH min-1 mgprot -1, 0.13 mmolNADPH min-1 mgprot -1, 0.17 mmolNADPH min-1 mgprot -1 and 0.21 mmolNADPH min-1 mgprot -1 at 50 mM, 100 mM, 150 mM and 200 mM of NaCl concentrations respectively (Figure 4E). GR activity increased by 320% at maximum NaCl concentration, i.e. at 200 mM (Table 1).
Discussion
Salinity stress is among the major abiotic stress affecting growth and development of plants. Various salt tolerant tree species have already been identified such as are Populus euphratica, Tamarix chinensis, Cocos nucifera, Avicennia marina, Pyrus betulaefolia, Rhus Typhina, etc are heavy salt tolerant tree species. Moderate salt tolerant tree species include Hippophae rhamnoides, Eucalyptus robusta, Pistacia chinensis, Gleditsia sinensis, Quercus rubra, etc. Light salt tolerant tree species are Jatropha curcas, Ginkgo biloba, Pistacia chinensis, etc. (Zhang, 2021). Identification of more salt-tolerant trees is an effective measure to increase the germplasm resources of salt-tolerant trees and improve saline soil management.
Simarouba amara is a multipurpose tree that is considered to grow well under different agro climatic conditions and on wastelandsKumar and Agrawal (2015). However, its ability to grow under salt stress conditions has not been studied yet. Present study was aimed to evaluate the effects of salt stress (NaCl) on morphology, antioxidants, lipid peroxidation and physiological activities in Simarouba amara DC. seedlings. In the current study, higher salt conditions (150 and 200 mM NaCl) proved to be toxic to S. amara seedlings in terms of morphology, leaf relative water content (LRWC), lipid peroxidation, and photosynthetic pigments. At higher NaCl concentrations, S. amara seedlings showed symptoms of leaf chlorosis, wilting, and drooping, which could be due to the accumulation of sodium ions in leaves in higher amount, affecting different physiological processes and causing ion toxicity. Presence of high toxic Na+ ions in the plants hampers the uptake of K+ ions, affecting stomatal conductivity, consequently leading to severe water loss, necrosis, etc. In the present study too, significant decrease in LRWC in S. amara seedlings was also observed under NaCl stress due to osmotic stress induction. Similarly, a decrease in LRWC was observed in Rosmarinus officinalis L. exposed to 50 and 100 mM NaCl concentrations (Mehrizi, 2012). Omami and Hammes (2006) also observed a decrease in LRWC in A. tricolor and A. cruentus plants exposed to PEG and NaCl treatments (separately and combined). Both of these stresses also resulted in decrease in leaf relative water potential in other studies (Kaydan & Yagmur, 2008) ; (Hassan, 2015).
Salt stress results in oxidative stress due to the formation of reactive oxygen species (ROS), which can affect the formation of important biomolecules like DNA, lipids, etc. Membrane lipid peroxidation is the major consequence of ROS, which is measured by calculating the malondialdehyde (MDA) content (). In our study, high lipid peroxidation was observed and MDA content significantly increased in response to increasing NaCl concentrations, clearly indicating ROS induced oxidative damage. Increase in lipid peroxidation with increase in the concentrations of NaCl has been observed in different studies (28); (29) ; (30) ;31 ; (32) ; (33).
Salinity stress also hampers the photosynthesis process and reduction in chlorophyll levels is generally observed due to oxidative stress. In the present study, photosynthetic pigments (chlorophyll a, chlorophyll b and carotenoids) decreased with increase in concentration of NaCl stress. Salinity stress reduces stomatal conductance and photosynthesis rate of plants (34) by changing various pigments concentrations (35) altering the organelles ultrastructure, stomata opening (36) and modifying different enzymes involved in photosynthesis process (35). Similar to our result, NaCl stress resulted in decrease in photosynthetic pigments in other studies also (37) ; (28) ; (33) ; (38) ; (39).
To mitigate the harmful effects of ROS, plants have active defensive system, comprising of enzymatic and non-enzymatic antioxidants. In the present study, amount of proline and cysteine increased significantly in NaCl treated seedlings, which reveals their protective role under stress conditions. Proline is an amino acid, osmolyte, and antioxidant molecule that is crucial for plants under high salinity stress conditions (radical scavenger). In the present study, proline content increased by 116% at maximum NaCl concentration (200 mM), showing its activity under stress conditions. Similarly, 40 observed percentage increase of 72.7% and 51.1% in proline content in Carthamus tinctorius L. cultivars, TSF1 and SM respectively, at maximum NaCl concentration, i.e. 2%.41 also observed 3.2 and 3.5 times increase in proline content in two rice varieties (Nonabokra and Pokkali respectively) at 200 mM NaCl concentration. Increase in proline content has also been observed in other study under NaCl stress conducted by42. Cysteine is a sulfur containing amino acid and an antioxidant that has ability to trap reactive oxygen species. In the present study, cysteine content increased significantly with an increase in NaCl concentrations. The role of cysteine in conferring protection against ROS damage has also been observed in different studies (43) ; (44) ; (45) .
Superoxide Dismutase (SOD: EC.1.15.1.1) is a metalloenzyme, that acts firstly to mitigate ROS toxic effects by breaking superoxide radicals into hydrogen peroxide. In the current study, activities of all the antioxidant enzymes significantly increased in response to NaCl stress. In the present study, SOD activity was found to be significantly increased with increasing salt (NaCl) concentrations. Our results are in corroboration with other studies in which SOD activity significantly increased in response to salt stress. In Lycopersicon esculentum,31 observed SOD activity of 25.56 U g-1 dm and 103.03 U g-1 dm in control plants and NaCl treated (100 mM) plants respectively. (30) also observed an increase in SOD activity in 45-day-old B. juncea and S. portulacastrum plants, when exposed to NaCl treatments.
Catalase (CAT: EC.1.11.1.6) is a heme-containing enzyme that breaks hydrogen peroxide into hydrogen monoxide and oxygen in peroxisomes. In the present study, CAT activity increased by 59% in response to maximum stress concentrations of NaCl (200 mM). Similar to our results,46 observed increase in CAT activity in leaves of salt sensitive and salt tolerant maize hybrids under NaCl stress. 47 also observed significant increase in CAT activity in two cultivars of T. aestivum L. (Alvand and Sardari) at low levels of NaCl, however at higher NaCl concentrations (200mM), catalase activity significantly decreased.48;49and50and also observed similar results of increase in CAT activity.
Ascorbate peroxidase (APX: EC.1.1.11.1) is a member of Ascorbate-Glutathione (ASC-GSH) cycle that dismutates hydrogen peroxide into hydrogen monoxide and Dehydroascorbate (DHA) in the cytoplasm and chloroplast. In the present study, APX activity also increased by 80% in response to maximum stress concentrations of NaCl (200 mM). Similar to our results, APX activity significantly increased under salt stress in other studies also.51 observed continuous and significant increase in APX activity in Mentha aquatica L. seedlings in response to NaCl stress at 50, 100, 150 mM concentrations. (46) also observed higher APX activity in leaves of two maize hybrids (26204 and 8441) at 100 mML-1 NaCl concentration compared to control. In another study conducted by 52 , % increase in APX activity at maximum NaCl concentration (120mM) in three Solanum species; S. stoloniferum, S. bulbosum and S. acaule was 330%, 162% and 169% respectively.
Guaiacol peroxidase (GPX: EC.1.11.1.7) is a heme-containing antioxidant enzyme that catalyzes the removal of extra hydrogen peroxide under normal and salinity stress conditions. In the present study, GPX activity increased by 80% at maximum concentrations of NaCl (200 mM) as compared to control. Similarly, GPX activity has also been found to be increased under NaCl stress in Carthamus tinctorius L. cultivars, TSF1 and SM (40) and rice varieties, Nonabokra and Pokkali (41) .
Glutathione Reductase (GR: EC.1.6.4.2) is a flavoprotein oxidoreductase that reduces oxidized glutathione (GSSG) into reduced glutathione (GSH) using NADPH. In the present study, GR activity increased by 320% in S. amara seedlings exposed to maximum concentrations of NaCl (200 mM). The increase in GR activity clearly indicating its role in mitigating ROS effects in S. amara seedlings. Our results are in corroboration with other studies in which GR activity significantly increased in response to salt stress.46 observed significant increase in GR activity in leaves of two maize hybrids (26204 and 8441) in response to NaCl, Cd and NaCl+Cd stresses.
Conclusions
Present study revealed severe lipid peroxidation, reduction in leaf relative water content and photosynthetic pigments due to toxicity of salt stresses at higher concentrations in S. amara seedlings. At higher salt concentrations (150 and 200 mM NaCl), S. amara seedlings also showed symptoms of leaf chlorosis, wilting, and drooping. However, in response to salt stress, activities of all antioxidant enzymes (SOD, CAT, APX, GPX and GR) significantly increased. Similarly, increase in concentrations of both non-enzymatic oxidants (proline and cysteine) was observed. A significant increase in the activities of both enzymatic and non-enzymatic antioxidants reveals that S. amara has better defensive mechanism against salt stress, but up to 100 mM concentration. At 150 and 200 mM NaCl, salt toxicity hampered growth of the plants. Present study could be very helpful in making strategies of S. amara large scale cultivation on wastelands particularly high saline lands.