Experimental Investigations on PVA: PVP Blend Based Nanocomposite Polymer Gel Electrolytes
Abstract
The aim of present work is to cast stable free standing PVA: PVP blend based polymer nanocomposite gel electrolyte films using ammonium acetate salt (CH3COONH4) and SiO2 nanofiller particles for electrochemical application. Optical and SEM studies show improvement in amorphous nature of the present system. Formation of blend-based nanocomposite polymer electrolyte is confirmed by FTIR study. Ionic conductivity of PVA: PVP blend based nanocomposite polymer gel electrolyte, namely, PVA: PVP: CH3COONH4:SiO2 is seen to improve moderately (~5 times) at room temperature with an optimum 5×10-3 S/cm for 8 wt % SiO2. I-t measurement (tion~0.9) establish dominance of ionic charge transport in synthesized electrolytes. Combination of Arrhenius and VTF behavior is reflected during temperature dependent conductivity analysis.
Keywords
PVA: PVP blend based electrolyte, SEM, FTIR, SiO2
Introduction
Polymer electrolytes have become materials of great importance for use in different electrochemical devices due to their unique properties like ease mouldability into any shape, light weight, flexibility adhesive property and good electrode- electrolyte contact. However, these polymer electrolytes exhibit low ionic conductivity in comparison to liquid electrolytes (Gray, 1991; Sekhon, 2003; Wieczorek, Florjancayk, & Stevens, 1995). Berthier et al established that crystalline phase is responsible for poor ionic conductivity in polymer electrolytes (Berthier, 1983). Over the years, various approaches (addition of plasticizer, use of copolymer, formation of polymer gel electrolyte etc) have been tried to augment the ionic conductivity to attain a value close to that of liquid electrolytes (Scrosati, 1992). Among these approaches, blending of two polymers leading to formation of blend-based polymer gel electrolyte has emerged as one of the attractive ways for conductivity improvement in polymer electrolytes (Xia, Soltz, & Samid, 1984). Therefore, blending of PVA with PVP was employed in this work. In general, PVA is solvent swollen character and known to form good hydrogels and also potential candidate for development proton conducting polymer electrolyte. Nature of PVP is semi crystalline and show good compatibility with PVA therefore, it is expected to improve the morphology and mechanical strength of polymer electrolyte. Most of the work on polymer gel electrolytes concerns to development of lithium- based polymer batteries (Caravanier, 2003; Koksbang, Oslen, & Shackle, 1994; Sato, Bonno, Maruo, & Nozu, 2005). Proton conductors have attracted much attention because of its potential use in clean energy devices such as fuel cell, electro chromic devices (ECD) and other smart devices (Colomban, 1992). Generally, polymer gel electrolytes possess good ionic conductivity near the liquid electrolytes but main drawbacks are poor dimensional, mechanical stabilities and liquid oozing from gel lump retained for a long period. To overcome these shortcomings composite electrolytes have been developed by incorporating the inorganic inert fillers. Addition of inorganic filler not only improves ionic conductivity but also enhances mechanical stability. In recent times, dispersion of in pristine polymer electrolytes (Agrawal et al, 2008) and in polymer blend based electrolytes (Mishra, Hashmi, & Rai, 2013) have been effectively tried to surmount these problems to a great extent leading to formation of nanocomposite polymer electrolytes. Looking into such problems, an attempt has been made in the present work to improve the performance of (PVA: PVP): CH3COONH4 electrolyte system by dispersal of nano- sized SiO2. This system is expected to drastically impede crystallization process in polymer-based nanocomposite electrolytes and thus improve ionic conductivity. These nanocomposite polymer electrolytes have been characterized using SEM, FTIR, ionic conductivity and electrical conductivity measurement to assess their electrical performance.
Experimental
PVA (average molecular weight 124,000- 186,000 Aldrich make), PVP (average molecular weight 360,000 Aldrich make), ammonium acetate (CH3COONH4), AR grade sd fine chem.and aprotic solvent dimethyl sulphoxide (DMSO) merk limited, were used for synthesis of polymer nanocomposite electrolyte. The preparation of nanocomposite electrolytes involved many steps. First both polymers PVA and PVP were dissolved in DMSO in the ratio of PVA: PVP, 80:20 and stirred for 3 hours at 500C to obtain homogeneous mixing.
In the second step an appropriate quantity of ammonium acetate (CH3COONH4) salt was added to the above mixture to get the composition so as to obtain the polymer blend electrolyte and stirred further for 2 hours to complete the homogenous mixing. In next step SiO2 nano filler was mixed in different wt% and stirred for other 2 to 3 hours.
The final solution was poured in poly carbonate petri dish to obtain thick films of nanocomposite electrolyte system. I-t measurements were performed on a CH- electrochemical workstation (CH instruments model CH-608) to determine electrochemical window and nature of charge transport. FTIR spectra were recorded on Nicolet iS10 spectrometer in range 4000-500 cm-1 to evaluate interaction among components. Electrical characterization of the material was carried out using impedance spectroscopy technique. Complex impedance parameters were measured with a Hioki impedance analyzer (model 3520) in the frequency range 40 Hz- 100 KHz using platinum electrodes for electrical contact.
Results and Discussion
Optical Studies
Figure 1(i) shows the optical micrographs of DMSO casted PVA, PVA: PVP blend and PVA: PVP blend based electrolyte while figure 1(ii) depict the view graphs for their nanocomposite electrolytes soaked with varying concentration of SiO2 nano filler. The optical image of PVA: DMSO shows porous structure (Chand, Rai, Agrawal, & Patel, 2011). When PVP is added in PVA to form PVA: PVP blend the pores seem to fade out. Upon addition of salt in PVA: PVP blend structure of blend is disturbed with appearance of enhanced pore density along with existence amorphous phase. Morphology of the film changes significantly upon addition of SiO2 {figure1 (ii)}. SiO2 filler nanoparticles are distributed randomly and attached on surface for lower concentration of nanofiller. Upon increasing the concentration of SiO2 filler particles pore size of electrolyte seems to be increase up to 8 wt%. Due to nano size of filler particles, the porous region of electrolyte is capable of holding agglomerates of nanofillers. This enhances the possibility of trapping of liquid electrolyte and thus overall electrical conductivity.
Scanning Electron Microscopy Studies
Figure 2 (a-d) depicts the surface morphology of DMSO casted PVA: PVP blend, PVA: PVP: CH3COONH4 electrolyte and its nanocomposite electrolytes containing 2 and 8 wt% of SiO2 nanofiller particles. White colored spots of about 1 μm are observed in the SEM image of PVA: PVP blend (figure 2 a) reflecting non mixing of polymer components due to incompatibility between polymers PVA-PVP system is known to exhibit limited compatibility (Grigoras & Barboiu, 2008). However, when salt is admixed in PVA: PVP blend to form blend-based electrolyte these white spots are reduced in size and amount which is a signature of improving compatibility between polymers thereby system moves to partial amorphous phase from crystalline phase (figure 2 b). When 2 wt% SiO2 nanofiller is introduced in blend-based electrolyte morphology of nano composite electrolyte film changes significantly. Few big and as well as uneven surface structure appears indicating entrapment filler by electrolyte matrix. Further these SiO2 nanofiller particles are distributed randomly and attached on surface (figure 2 c). On addition of 8 wt% of SiO2 nanofiller matrix of polymer electrolyte is fully covered with SiO2 nano particles and layered structure is noticed (figure 2 d). This indicates complete dispersion of SiO2 nanofiller in electrolyte film. Owing to coverage of porous region of film with nano particles there is possibility of trapping of liquid electrolyte coercing enhancement in electrical conductivity.
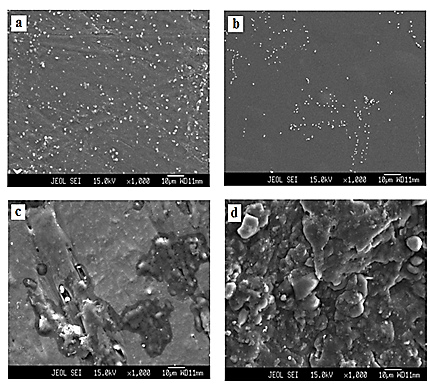
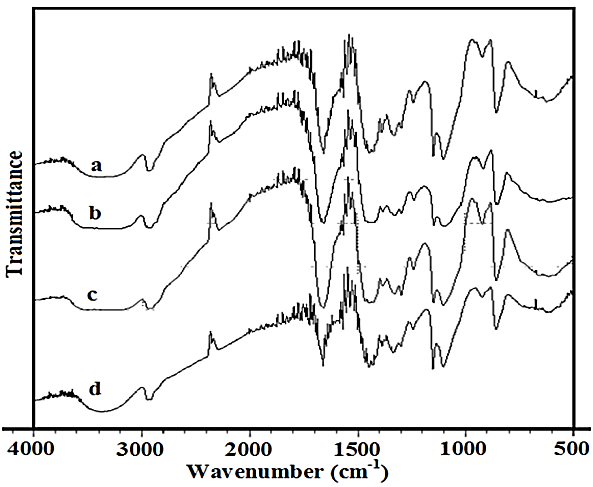
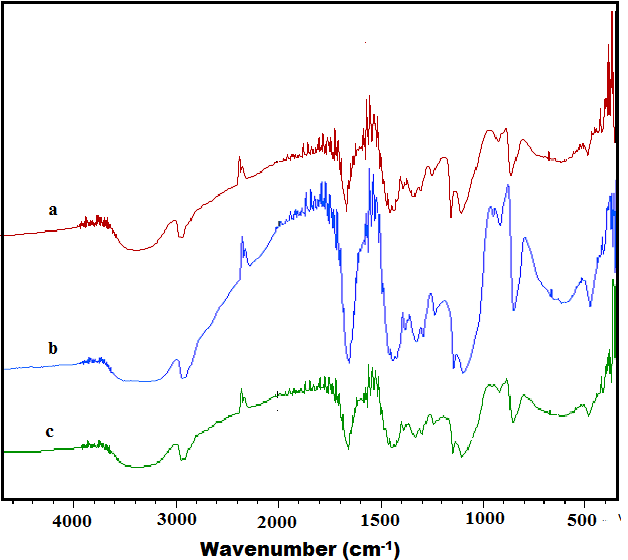
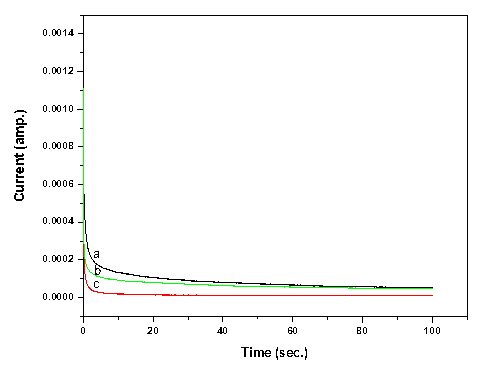
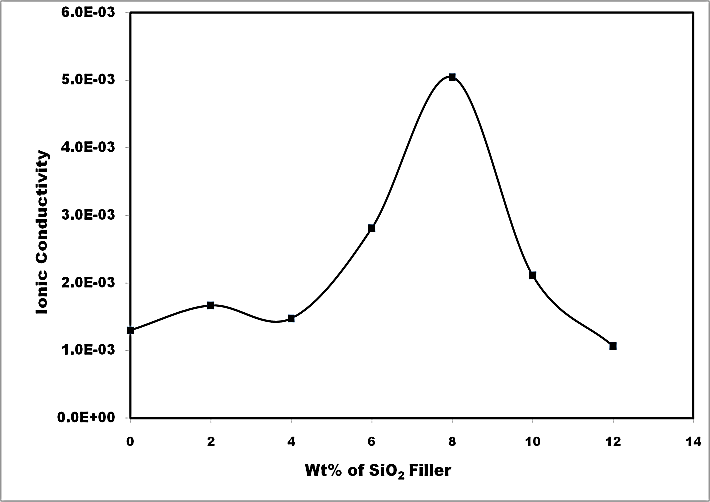
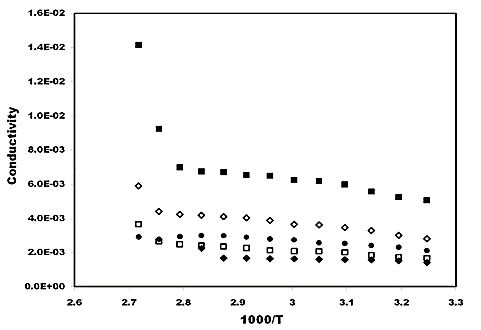
FTIR Studies
Wagner’s Polarization Studies
The total ionic transport number (tion) was evaluated using the well-known d.c. polarization technique to assess the nature of charge transport (Hashmi & Chandra, 1995). In this technique, as synthesized blend nanocomposite electrolyte samples were polarized by applying a step potential across cell configuration SS| electrolyte |SS and the resulting potentiostatic current measured as a function of time. The variation of current with time for few electrolyte samples is shown in figure 4. Polarization behavior is reflected in all these curves as per expectation. The initial current initial and final current ifinal was noted from the polarization curves and the total ionic transference number tion was calculated using following relation:
= (1)
The calculated values of tion for different composite films varies from 0.89 to 0.99 with varying molar concentration of salt and filler content. Such large values of total ionic transference number ascertain predominance of ionic charge transport over electronic transport in polymer blend based electrolytes.
Electrical Conductivity
Figure 5 depicts the variation of ionic conductivity of blend-based polymer nanocomposite electrolyte with SiO2 filler concentration. The ionic conductivity of nanocomposite electrolyte is seen to improve moderately (~5 times) at room temperature for 8 wt% of SiO2 filler. However, close observation of conductivity plot shows two maxima, one around 2 wt% and another around 8 wt% filler concentration- a feature typical of nanocomposite polymer electrolyte (Chand et al., 2011). Enhancement in ionic conductivity is noticed to be of small magnitude at low filler concentration (2 wt% SiO2). This feature can be associated to the fact that all the salt has been dissociated and so charge carrier concentration is limited. It is only the change in system morphology that tends to affect the conductivity as also evidenced in XRD studies. In situations, when there is large quantity of nanoparticles (in excess of 4 wt% SiO2) a sharp rise in room temperature conductivity takes place and attain an optimum for electrolytes containing 8 wt% filler. This might be due to better compatibility among components leading to re-dissociation of salt into respective free ions giving rise to significant increase in ionic conductivity. Beyond 8 wt% SiO2 concentration a significant fall in ionic conductivity is seen. This result from agglomeration of these nanoparticles which tend to create tortuous pathways for mobile ions and thus conductivity drops. Another factor that can be associated with the fall in conductivity is the increase in the microscopic viscosity of composite system as envisaged in breathing chain model (Chandra, Sekhon, & Arora, 2000).
Temperature Dependence Conductivity
Fig 6 depicts the temperature dependence conductivity of blend-based polymer nanocomposite electrolytes at different wt% of SiO2 nano filler particle. The variation of conductivity is seen to change slowly with temperature. At low temperature (around room temperature) the conductivity response is essentially dictated by the trapped liquid electrolyte within the polymer matrix and hence Arrhenius behavior is noticeable. However, as the temperature approaches the glass transition temperature of polymer PVA (75°C) in polymer composite system, the matrix becomes flexible giving rise to change in conductivity values. The enhancement in conductivity with increasing temperature can be rationalized with free volume model (Miyamoto & Shibayama, 1973). The behavior of blend-based electrolyte and their nanocomposite of different wt% of SiO2 nano filler can be described by combination of Arrhenius and VTF relation.