Investigation On Nanocomposite Polymer Gel Electrolyte Films And Their Nanofibers For Electrochemical Devices
Abstract
Present work has been aimed to study the role of MWNT on the performance of a PVA based nanocomposite polymer gel electrolyte films and their nanofibers, namely, {(PVA:NH4SCN):MWNT} system. The films and fibers, prepared by solution cast method and electrospinning respectively have been characterized by structural, thermal, electrical and cyclic voltametry measurements. A relative examination of XRD of nanocomposite polymer electrolytes (NCPEs) systems based films and fiber mats show that fibers exhibit better amorphous behavior in contrast to film counterpart. DSC results on fibers show greater thermal stability in comparison to corresponding gel electrolyte films. Likewise Cyclic Voltammetric investigations on fibers mats have better electrochemical stability viz. ±1.6 volt. However, bulk conductivity data for NCPE fibers mats exhibit comparable ionic conductivity. All these results indicate fiber mats as an alternative to electrolyte films for application in electrochemical devices.
Keywords
Polymer gel electrolytes nanofibers, X Ray Diffraction, Differential scanning calorimetry and conductivity
Introduction
Over the years, polymer electrolytes have emerged as potential candidate for various electrochemical devices (such as high performance batteries, fuel cell, super capacitor, sensors, smart window etc.) on account of possibility of achieving high ionic conductivity, better mechanical, thermal stability and the ability to form proper electrode-electrolyte contacts (Adebahr, 2003) (Awadhia, 2007); (Chandra, 2000) (Colomban, 1992); (Owen, 1989); (Suthanthiraraj & Sheeba, 2007). Within this class polymer gel electrolytes are known to suffer from mechanical degradation and solvent exudation despite their high ion transport behavior. Researchers in recent times have dispersed non-interacting filler particles like SiO2, Al2O3, TiO2, Ba ferrite etc. to over-come these drawbacks thereby creating a new class of polymer electrolytes, termed as, nanocomposite polymer gel electrolytes (NCPGE). Among the favorable polymer host for synthesis of nanocomposite gel electrolyte, PVA is a semi crystalline, water soluble polymer with 1, 3, glycol structure where in anion / cation mobility occurs in the amorphous phase and its diffusion occurs through a complex mechanism involving PVA segmental mobility. Moreover, this polymer contains hydroxyl groups attached to methane carbons which are active sites for complexation with different salts and acids. Owing to possibility of achieving maximal ionic conduction with protons/NH4 + ions as transporting specie in gel electrolyte systems, it has been used as a host for development of proton conducting electrolytes in recent past (Shukla, 2000). Further, it possesses relatively low glass transition temperature and high melting temperature and has an exceptional property to dissolve high concentration of a wide variety of dopants. Prompted by these considerations, attempts have been made in the present work to develop a proton/ammonium ion conducting nanocomposite polymer gel electrolyte based on host polymer PVA. Among the different dopants materials, NH4SCN salt has been considered as a favorable solute owing to small cationic/bigger anionic size which allows greater mobility for transporting ion. Among the different nanofillers used in development of nanocomposite system, multi walled carbon nanotube (MWNT) which possess unique mechanical properties and usually characterized by high thermal stability, have off late been suggested as effective active dispersoid material for development of NCPGEs. Such nano material based systems exhibit better thermal and electrochemical behavior and conductivity as well. An innovative approach has been made in the present work to synthesize nanofibers of these composite membranes through electrospinning technique as well. The NCPE gel membranes and fibers of PVA-NH4SCN system dispersed with MWNT were also characterized through different experimental tools like XRD, DSC, Impedance spectroscopy and Cyclic voltametry to assess their performance.
Materials and Methods
PVA (average molecular weight 124, 000–186, 000, Aldrich make), ammonium thiocyanate (NH4SCN), AR grade, and aprotic solvent dimethyl sulphoxide (DMSO) were used for synthesis of composite gel films and fibers. MWNT nanoparticle used in the study was obtained from Aldrich, CAS Number: 308068-56-6, possessing average diameter 70-110nm and length 6-9 μm. PVA (5wt%) was dispersed in 1M salt solution of NH4SCN in DMSO to form pristine gel electrolyte (PVA: NH4SCN system). Subsequently, Composite polymer gel electrolyte viscous solutions were prepared by admixing MWNT in pristine gel electrolyte solution in different weight proportions followed by thorough mixing at slightly elevated temperature on a magnetic stirrer for ten hours. To obtain free standing NCPGE films one portion of so formed solutions were poured in PC pettridishes and covered with Al-foils to avoid contamination. After synersis, gels in the form of thick stable gel films were taken out. Fibers were prepared using electrostatic spinner coupled with a high voltage dc power supply. In the experiment, dc voltage was set at 22 kV while the distance between the spinneret and the fiber collector was mechanically adjusted at 14cm to achieve good quality of fibers at room temperature (28°C). The second portion of gel solutions as described above were filled in the syringe and a voltage of 22 kV was applied to the gel solution (figure 1).
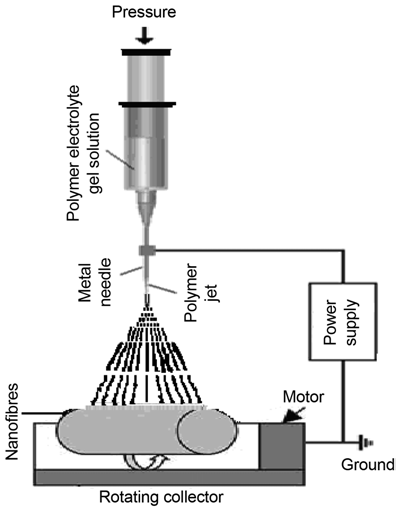
A fiber mat was formed over aluminium foil and collected for further study. Synthesized NCPE gel films and their nanofibres have been characterized using different experimental probes as below.
Structural morphology of different nanocomposite polymer gel electrolyte films and fibers were studied by X-ray Diffractometer (model no. RIGAKU JAPAN MINIFLEX-II). The diffraction pattern was recorded at room temperature (~25˚C) using Cu-Kr target (λ=1.503 Å) for the Bragg angles (2θ) varying from 2˚ to 90˚ with the scan speed of 1˚min-1.
Thermal measurements were performed on a Mettler Toledo make Differential Scanning Calorimetry (model 822e). Thermal scan of samples were recorded in open-air environment at a heating rate of 10°C/min. in the temperature range 40-240°C to assess their thermal behaviour.
The complex-impedance measurements were carried out with help of a computer controlled Hioki (Japan) make LCR meter (Model 3520). After sandwiching the NCPE gel films and fiber mats between two platinum electrodes, electrical conductivity measurements were performed in the frequency range varying from 40Hz–100 kHz at various temperatures ranging between 25˚C and 100˚C.
Cyclic Voltammetry analysis was carried out in air at room temperature using an Electrochemical Analyzer (CH Instruments, USA model no. CHI608D) in the voltage sweep range ±3V keeping the scan rate at 0.1Vs-1 to assess electrochemical stability of samples and electrochemical window.
Result and Discussion
X Ray Diffraction Studies
The XRD patterns of polymer electrolytes gel films and fibers of DMSO:NH4SCN:PVA without and with Al2O3 nanofillers along with pristine materials are shown in figure 2 (a-h). The XRD (pattern a) comprises of characteristics strong peak of MWNT at 2θ ~ 26.2°, 42.4°, 54.1°, 77.5° and it shows pure crystalline structure. Diffractogram (b) corresponds to ammonium thiocyanate displaying intense and distinguishable peaks at 14°, 18°, 25.4°, 30.7°, 33.6°, 34.3°, 37.8° and 38.7° on 2q scale, conforming to its monoclinic structure (Cullity, 1978). The XRD pattern for PVA:DMSO:NH4SCN film (pattern d) exhibits shifting of PVA related peak with reference to PVA:DMSO film (pattern c) towards lower 2θ value (17.5º) on addition of salt NH4SCN in PVA matrix. Additionally, a shoulder peak at 2θ=15° appears in PVA-NH4SCN electrolyte. Both peaks do not match to either salt or polymer and hence corroborates the formation of a new material viz. PVA-NH4SCN complex indicating complete solvation/complexation of salt in gel matrix (Saikia & Kumar, 2004). On addition of 6 wt% MWNTs content (pattern e), the intense peak (17°) and shoulder peak (14°) are seen to shift to higher 2θ value (18° & 15° respectively) along with increasing broadness and significant loss of intensity. Addition of MWNT prevents polymer chain reorganization, which causes significant disorder in the polymer chains and this promotes interaction among components leading to enhancement in amorphous behavior of PNCGEs. This feature ascertains improvement in system morphology. In case of nanofiber mats of the samples similar behavior is obtained except broadening of peaks at 2θ values (pattern f). Further no other peak related to MWNT could be tracked in the composite nanofibers to suggest the role of MWNT as that of passive filler.
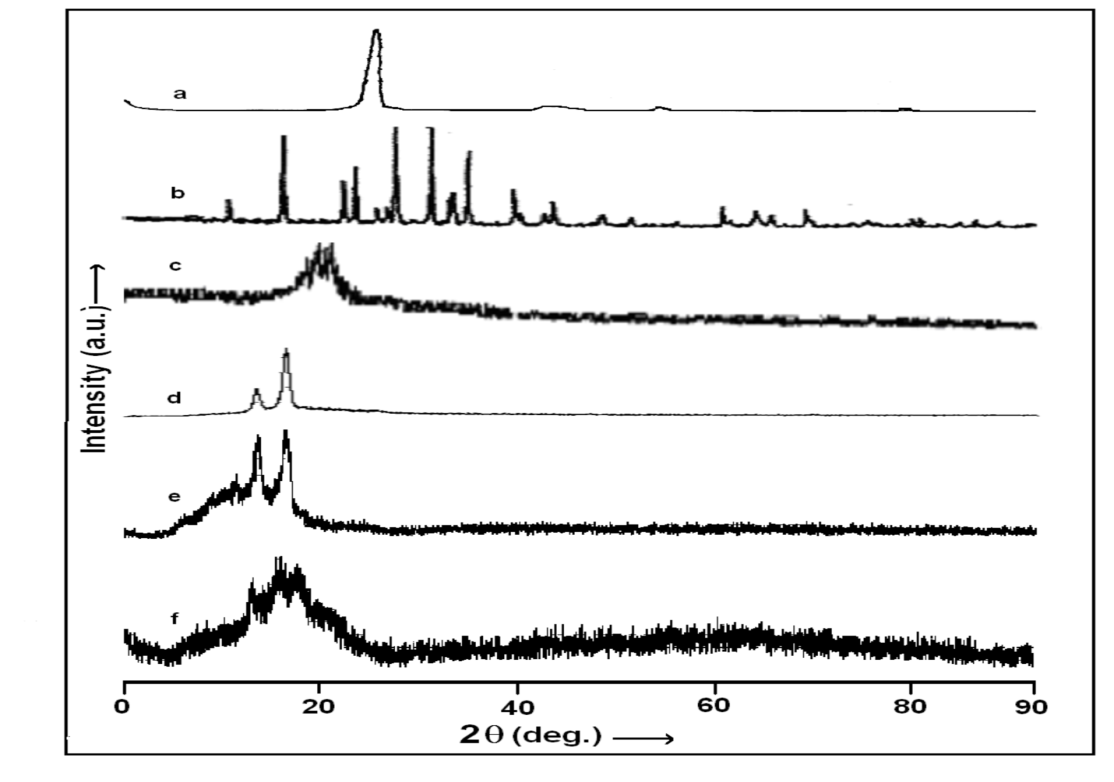
The XRD (pattern a) comprises of characteristics strong peak of MWNT at 2θ ~ 26.2°, 42.4°, 54.1°, 77.5° and it shows pure crystalline structure. Diffractogram (b) corresponds to ammonium thiocyanate displaying intense and distinguishable peaks at 14°, 18°, 25.4°, 30.7°, 33.6°, 34.3°, 37.8° and 38.7° on 2q scale, conforming to its monoclinic structure (Cullity, 1978). The XRD pattern for PVA:DMSO:NH4SCN film (pattern d) exhibits shifting of PVA related peak with reference to PVA:DMSO film (pattern c) towards lower 2θ value (17.5º) on addition of salt NH4SCN in PVA matrix. Additionally, a shoulder peak at 2θ=15° appears in PVA-NH4SCN electrolyte. Both peaks do not match to either salt or polymer and hence corroborates the formation of a new material viz. PVA-NH4SCN complex indicating complete solvation/complexation of salt in gel matrix (Saikia & Kumar, 2004). On addition of 6 wt% MWNTs content (pattern e), the intense peak (17°) and shoulder peak (14°) are seen to shift to higher 2θ value (18° & 15° respectively) along with increasing broadness and significant loss of intensity. Addition of MWNT prevents polymer chain reorganization, which causes significant disorder in the polymer chains and this promotes interaction among components leading to enhancement in amorphous behavior of PNCGEs. This feature ascertains improvement in system morphology. In case of nanofiber mats of the samples similar behavior is obtained except broadening of peaks at 2θ values (pattern f). Further no other peak related to MWNT could be tracked in the composite nanofibers to suggest the role of MWNT as that of passive filler.
DSC Studies
The DSC curves obtained for various NCPGEs gel films and their nanofibers are represented in figure 3 (a-f). PVA is known to be partially crystalline material and so both characteristic transitions are likely to appear in DSC thermogram. The glass transition temperature and melting temperature of PVA are seen to occur at 88°C and 216°C respectively (scan b).A broad shoulder peak around 170°C is also witnessed in the DSC profile of pure PVA. Such a feature has also been reported earlier (Zou, 2008), and ascribed to phenton melting (Mukherjee, 2004). The melting endotherm (curve a) of highly crystalline ammonium thiocyanate is obtained at 148°C (Awadhia, 2007) (Awadhia & Agrawal, 2007). In consonance with earlier reports (Shukla, 2000) , pristine electrolyte thermogram (Scan c) depicts two endothermic transitions including two small shoulders related to phenton melting (~160ºC) and uncomplexed PVA respectively (around 200°C). The strong endothermic peak observed around 168°C is linked to melting of gel mass of PVA:DMSO:NH4SCN complex and partially uncomplexed PVA:DMSO gel which may have different conformations of complexes. Occurrence of multiple peaks indicates heterogeneous distribution of complexes.On adding 6wt% of MWNT nano particles in pristine gel electrolyte to form composite gel electrolyte, an interesting feature is observed that initially no transitions are apparent till 200°C indicating increase in thermal stability of NCPE. Similarly, in case of MWNT filled polymer electrolyte fiber mats (scan e&f), the DSC thermograms didn’t reveal any significant change in thermal transitions though slightly decreased the melting temperature of uncomplexed PVA. This is possibly due to change in chain entanglement/change in chain length of polymer in the presence of high electric field used in preparation of nano fibers. Further insignificant change in enthalpy (ΔH~0) till 220ºC suggests completely amorphous behavior of synthesized electrolyte fiber. It is concluded from these investigations that electrostatic spinning produces completely amorphous electrolyte nanofibers with large thermal stability comparatively gel films.
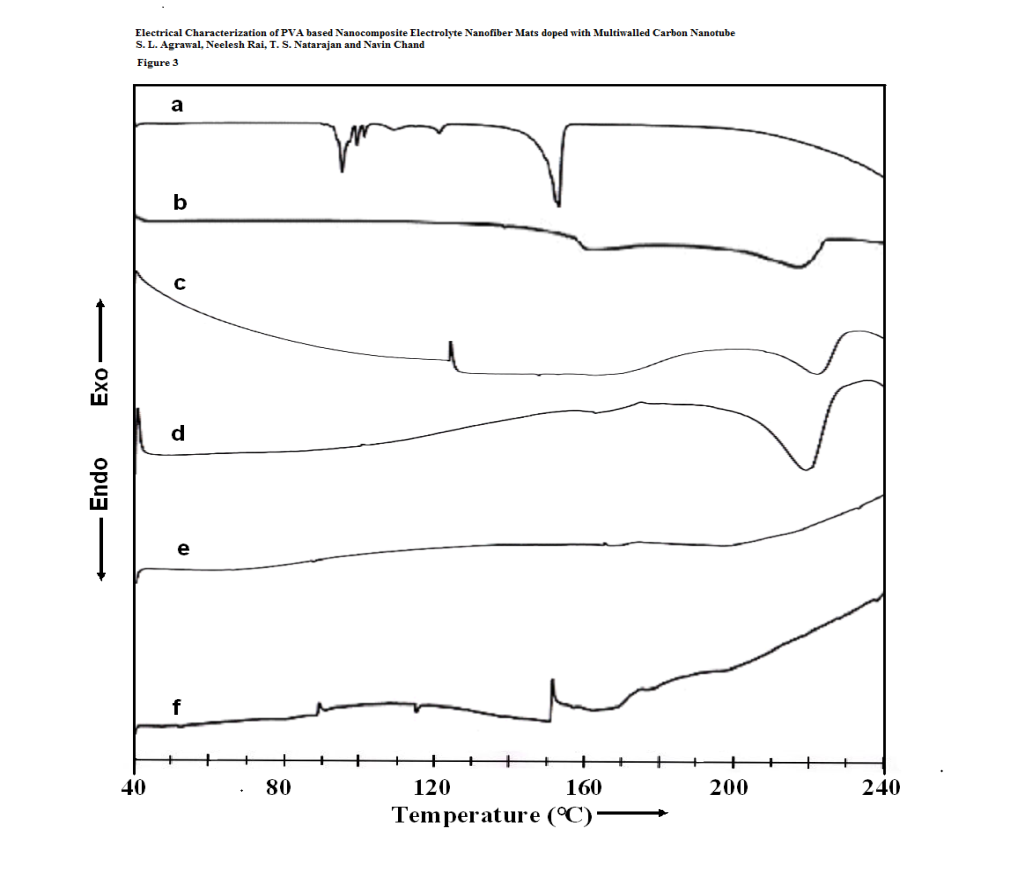
Electrical Conductivity
Figure 4 shows the role of MWNT nano particles concentration on ion conduction behavior of polymer gel electrolytes. In the presence of filler, conductivity is seen to rise by roughly an order of magnitude. Closer examination of conductivity behavior shows two maximas one around 3 wt% and another around 6wt% filler concentration - a feature typical of polymer nanocomposite gel electrolytes (Pandey, 2008). A flattening in conductivity response is noticed beyond 3wt% MWNT contents. This can be associated to the fact that all the salt has been dissociated and so charge carrier concentration is limited. It is only the change in system morphology that tends to affect the conductivity and thus again an enhancement of small magnitude is visible. Flattening of bulk electrical conductivity is restricted upto 4wt % MWNT content and there after it again shows an enhancing behavior till 6wt% filler.
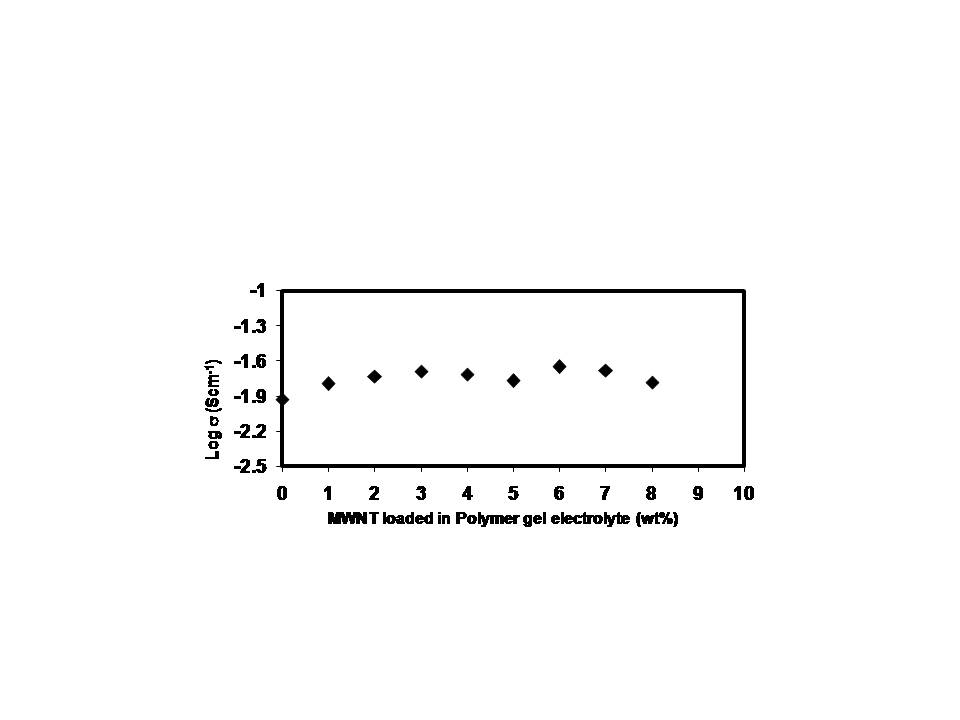
This rise in conductivity at intermediate filler concentration (i.e. beyond 4wt%) is on account of dominance of system morphology over the decreasing trend in the number of transporting ions causing a small increase of conductivity. Another explanation to the rise in conductivity can be provided in terms of the breathing chain model proposed by (Chandra, 2000). According to this model ion dissociation results from folding and unfolding of the polymeric chains which creates free volume due to local pressure fluctuations for the ions to migrate easily. This overcomes the decreasing trend of transporting ions to again help in escalating ionic conductivity as seen in figure 4a. However, in situations when there is very large quantity of nano filler (beyond 6wt%) present in composite system, agglomeration of these nano fillers takes place which leads to decrease in conductivity due to creation of tortuous pathways for mobile ions. Another factor that can be associated to fall in conductivity beyond 6 wt% filler content is the increase in microscopic viscosity of the composite system. Thus, an optimum in conductivity was found (5.81x10-2 S/cm) for 6 wt% of MWNT. Figure 5 depicts variation of ionic conductivity of electrolyte nanofibers as a function of MWNT concentration. Contrary to the two conductivity maxima observed for electrolyte films (figure 4a), conductivity behavior of electrolyte fibers show a gradual increase with filler concentration and it tends to saturate though the change remains within an order of magnitude. The slight improvement in conductivity is due to increase in amorphous structure of fiber mats as noticed during XRD and DSC studies. Existence of improved amorphous phase tends to increase conduction pathways for ion transport. Another possibility may be an increase in conduction pathways in the presence of MWNT nano filler (Adebahr et al., 2003). Behavior of MWNT dispersoid as passive nanofiller has been affirmed during XRD and DSC investigations.
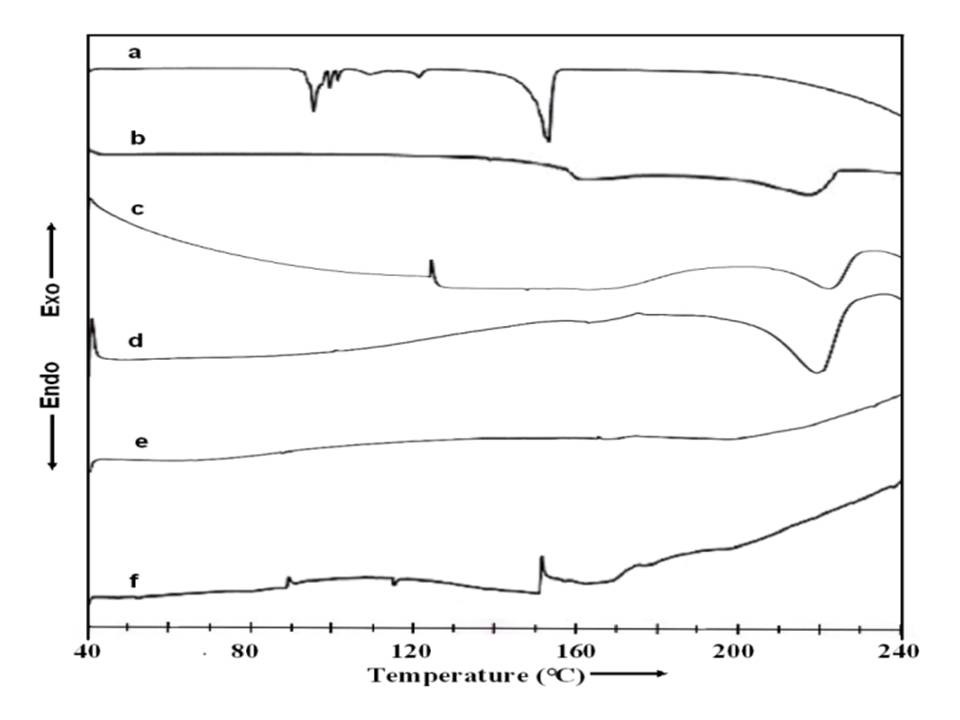
The conductivity value obtained for PVA:NH4SCN:MWNT electrolyte fibers is slightly lower than the corresponding result for gel membranes prepared by solution cast technique (Awadhia & Agrawal, 2007). This observation can be associated to significant drying of samples/stretching of chains in the presence of high electric field. In polymer electrolytes, the ionic conductivity has been shown to depend upon the number of free charge carriers and improved morphology of the system (Awadhia & Agrawal, 2007). In the present investigations, the enhancement in electrical conductivity in electrolyte fibers can be ascribed to the porous structure of electrospun membrane and improvement in morphology as witnessed in XRD and DSC studies which allows greater absorption of NH4SCN salt and filler within the fiber.
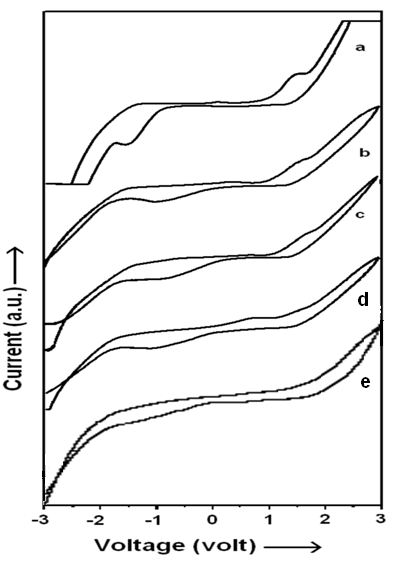
Cyclic Voltametry Studies
Figure 6 (a-e) shows the cyclic voltagrams for pristine electrolyte and nano MWNT doped PVA:NH4SCN:DMSO composite electrolytes gel films and their nanofibers. It is apparent from this figure that electrochemical window extends from -2.5 to +2.5 volt for pristine electrolyte (figure 5a) and -3.0 to +3.0 for NCPGEs {figure 5 (b-d)} under investigation. Small cathodic and anodic peaks were also observed around ±1.3V in pristine electrolyte (scan a).
The appearance of these peaks is essentially due to oxidation/reduction of trapped solvent (DMSO) in the polymer electrolyte as also evidenced in XRD investigations and cyclic voltagram of solvent DMSO. These anodic and cathodic peaks diminish in intensity and become broad along with shift of anodic peak towards higher potential and cathodic peak towards lower potential in each of the voltagram of NCPGEs (scan b-d). This is essentially due to interaction of filler with the NH4SCN salt in the presence of DMSO. An interesting observation is the appearance of a single oxidation peak observed around +0.5V for all pristine and composite gel electrolytes which is possibly on account of NH4 +/H+ ion that contributes to ionic conduction. Such an observation has been previously reported for PEO based NCPE (Agrawal, Singh, Dwivedi, Tripathi, & Pandey, 2009); (Bhargav, Mohan, Sharma, & Rao, 2007). Stability is seen to improve significantly (-2V to +1.7V) and NH4 + ion related peak could not tracked in cyclic voltagrams of electrolyte nanofibers with 6wt% MWNT filler in contrast to its gel electrolyte due to loss of DMSO related oxidation/ reduction peak value during electrospinning at high electric field (scans e). This feature ascertains improvement in system morphology and electrochemical stability nanocomposite electrolyte fibers in the presence of MWNT fillers. A stable potential window is of great practical importance for applications in batteries and super capacitors (Bhargav et al., 2007; Kubota et al., 2002).
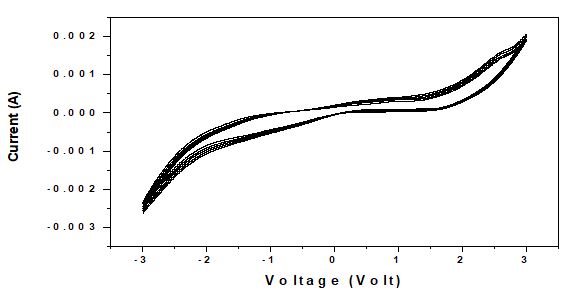
Ionically conductive polymer gel electrolytes consisting of crosslinked methacrylonitrile and organic electrolyte. In view of this repeated cycle measurements of cyclic voltametry were performed for one of the samples i.e. 6wt% loaded PVA:NH4SCN:MWNT nanocomposite fibers (figure 6). It is observed that the area under the curve do not decrease appreciably ever after 05 cycles which indicates that fibers are stable and can be used in device applications.
CONCLUSION
Present comparative investigations reveal that developed nanocomposite polymer electrolyte nanofibers containing MWNT nanofiller is the best ion conducting system having better morphology and high ionic conductivity. Enhanced amorphous behavior is revealed in case of fiber mats from XRD studies of NCPEs systems based gel films and its corresponding fiber mats. DSC results of fiber mats show higher thermal stability as compared to corresponding gel electrolyte films. Bulk conductivity data shows best response for fiber mats. Cyclic Voltammetric investigations on fibers mats have shown that better electrochemical stability viz. -2V to +1.7V. Current examinations thus suggest electrospinning of fiber mats of nanocomposite polymer gel electrolyte as a suitable technique of achieving thermally, electrochemically and mechanically stable electrolytes with ion conductivity properties approaching that of liquid electrolyte. Such properties are expected to open up new application areas particularly in electrochemical devices.
ACKNOWLEDGEMENT
The author wish to acknowledge to AMPRI (CSIR) Bhopal for providing DSC measurement facility and Department of Physics, IIT Madras for providing electrospinning setup. I also would like to thanks Prof. S. L. Agrawal, APS University, Rewa for giving facility to perform electrochemical measurements.
CONFLICT OF INTEREST
The author declares no conflict of interest.