Crosstalk-interaction of Nitric oxide in plant growth and development under abiotic stress
Abstract
Nitric oxide (NO) is endogenously produced short-lived free radicle and dispersed inside plant cells where it takes part in the multi-functional physiological role under optimal as well as biotic and abiotic stress. Under harsh conditions, NO modulates the antioxidant system, maintain osmotic balances, and regulates gene expression by different post-translation modification (PTM). Dose-dependent role of NO to ensure plant survival under adverse condition is well-known but still need précised and scientific research to delineate the underlying mechanisms, which are not clearly known. The endogenous level of NO is also an important parameter to mitigate the negative effect of abiotic stress which is directly or indirectly depend upon the involvement of other growth regulators. The multidimensional role of NO under salinity, drought, heavy metal toxicity, and the extreme temperature has also been discussed with update research available till date for better understanding. In this review, an effort has been made to talk about the portion subordinate job of NO in plant development and the advancement process under the ordinary and ideal procedure. Moreover, the role of NO in major abiotic stress and its cooperation with significant plant development controller has additionally been critically discussed.
Keywords
Nitric oxide, Reactive oxygen species, Abiotic stress, Phytohormones
Introduction:
Nitric oxide (NO), is free radical diatomic gaseous molecule that diffuses across the membrane and acts as a signaling regulator and considered as “Molecule” of biological importance (Domingos, Prado, Wong, Gehring, & Feijo, 2015). Initially, NO was discovered in the mammalian system, research was mainly focused on its signaling role in the animal, but in the late 1990s, NO gained attention for its importance in plant immunity against various pathogens. Besides as signalling molecule, NO coordinate and regulates growth and development of plants, from germination to flowering stages (Sami et al., 2017; ) (Fatima, Husain, Suhel, Prasad, & Singh, 2022). NO-directed post-translation modification such as metal nitrosylation, S-nitrosylation, tyrosine nitrations governed the regulatory action in plant growth under normal and harsh situations (Prakash et al., 2020). The excessive formation of reactive oxygen/nitrogen species in a plant cell is the initial cause of stress conditions (Ahmad et al., 2016). NO scavenges ROS and modulates the antioxidant system directly by regulating the endogenous level of NO and or its crosstalk between NO and ROS and different plant growth regulators by mitigating nitro and oxidative stress (Hasanuzzaman et al., 2017). Apart from these abiotic stresses, plants demonstrated systemic acquired resistance (SAR), which induces a hypersensitive response (HR) initiated by NO and improves plant immunity against bacterial, microbial, and fungal pathogens (Yu, Lamattina, Spoel, & Loake, 2014). NO triggered a particular signal cascade and caused programmed cell death (PCD) when tolerance was not induced under biotic and abiotic stress conditions (Groß, Durner, & Gaupels, 2013). Despite much improvements in biochemical and molecular technology, NO-mediated stress, which is caused by scavenging ROS levels, activating the defense framework, accumulating osmolytes, and interacting with and stimulating various hormone-interceded signaling responses, has not yet been fully understood and requires further advanced research using a detailed mechanistic approach. The multifaceted role of NO and its interactions with other plant growth regulators under various abiotic stressors, such as salinity, heavy metal toxicity, and drought, will thus be the main emphasis of this review.
Dose-dependent role of NO in growth and metabolic process
NO regulates growth and physiological processes such as apical meristem growth, lateral growth formation (Gupta, Devi, & Singh, 2022; Wen et al., 2016), embryo development, somatic tissue development, cell cycles, stomatal movement (Domingos et al., 2015; Hebelstrup, Shah, & Igamberdiev, 2013), floral development (Zafra, Rodríguez-García, & Alché, 2010) and fruit ripening. The beneficial effects of NO are dose-dependent and may differ from crops to crops. For example, lower concentrations improved growth parameters in tomato and pea plants, whereas higher concentrations inhibited growth in both crops. (Hayat, Yadav, Wani, Irfan, & Ahmad, 2011). Furthermore, a lower concentration of SNP (sodium nitropurside) promotes root growth (maize), leaf expansion (pea), and seed germination (tomato, brassica) (Tian & Lei, 2006), whereas a higher concentration inhibits all of these processes. NO promotes adventitious root formation by activating MAPK (Mitogen-activated protein kinases) and cell cycle gene(s) (Fernández-Marcos, Sanz, & Lorenzo, 2012). Lower NO concentrations increased photosynthetic rate, chlorophyll content, and stomatal conductance, but SNP (dose-dependent) showed an inhibitory effect of Rubisco activity by S-nitrosylation (Abat, Mattoo, & Deswal, 2008). Exogenous application of SNP improved and increased the content of photosynthetic pigments while also preventing chlorosis in Fe-deficient mesophyll cells of Zea mays (Sun et al., 2007). NO is also associated with pollen tube growth and positioning, but the full mode of action is dependent on cGMP concentration, which in the absence or low concentration failed to achieve proper orientation (Sami et al., 2018). On the contrary, it was discovered that a low concentration or accumulation of NO near the tip inhibits growth and orientation; the reason for this is unknown (Prado, Colaço, Moreno, Silva, & Feijó, 2008). Flowering is an important and productive phase of the plant life cycle that requires specific gene expression, such as the photoperiod transcription factor CONSTANS (CO), which is repressed by the NO-mediated-post-translation modification factor to delay flowering. NO- mediated S-nitrosylation was achieved by suppressing the gene (LEAFY) and increasing the expression of FLC (FLOWERING LOCUS C), a key repressor of flowering (Astier et al., 2011). It has also been reported that during the normal life cycle of a plant, a shift in Ca2+ concentration indicates a signal sensed by calmodulin (CaM), and that conformational changes promote the expression of CO and initiate early flowering (Tsai et al., 2007). This mechanism is absent in the mutant line, and flowering is delayed as a result of increased concentrations of endogenously produced NO (Tsai et al., 2007). Recently, a sequential decrease in NO concentration in senescing pea plant leaves and downregulation of NOS activity was observed in peroxisomes (Rio et al., 2004). Schematic representation of the significant functions mediated by NO has been shown in Figure 1.
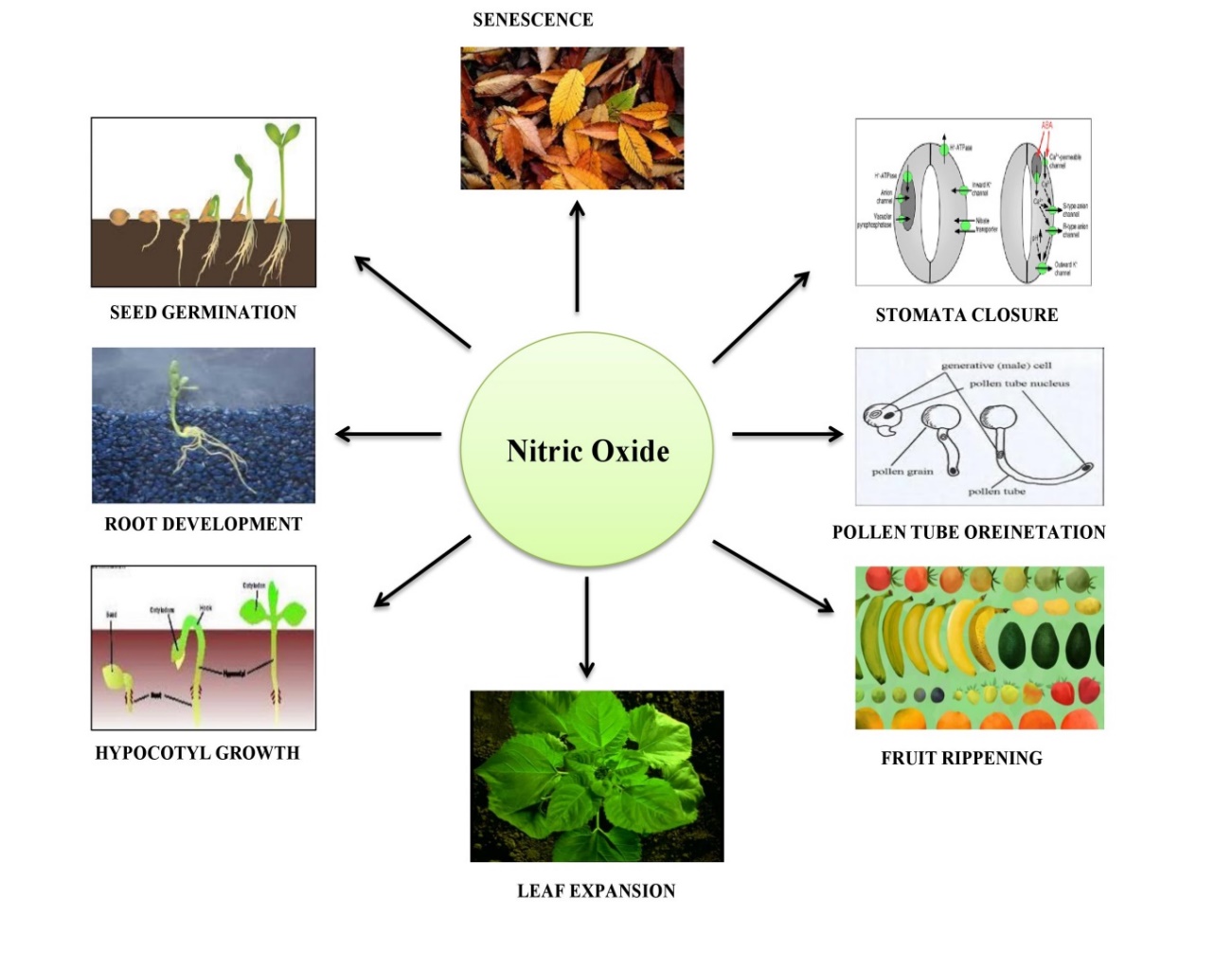
Nitric oxide-mediated modulation of plant antioxidant defense system under major abiotic stress
In the modern epoch, rapid urbanization, extreme climatic changes, excessive usage of hazardous chemicals (pesticides) significantly reduced agricultural productivity worldwide (Kamal et al., 2020). The problem is intensified by various abiotic stresses such as water scarcity (drought) or salt and ion accumulation in soil making water unavailable to plants (salinity), extreme temperature (heat, cold, chilling stress), massive accumulation of toxic metal(s) (heavy metal toxicity), low and high intensity of light and radiation (Ahmad, Singh, & Kamal, 2019; Zhang, Zhu, Gong, & Zhu, 2022). However, the intensity and impact of these abiotic stresses vary depending on plant species and duration, but the ultimate consequences include a rapid increase in ROS levels (O2; OH; HO2; RO; H2O2, 1O2) in different organelles of the cell, which ruled out antioxidant defense mechanisms by disrupting the equilibrium of redox homeostasis (Gill & Tuteja, 2010) (Figure 2). ROS signals cause oxidative stress, which leads to the degradation of biologically active molecules such as nucleic acid, protein, and sugar molecules, halting the cellular machinery and causing cell death (Gill and Tuteja (2010)Gill and Tuteja (2010); Anjum et al., 2014). NO efficiently detoxify ROS by activating antioxidant enzymes at the cellular and organelle levels (mitochondria, chloroplasts, and peroxisomes) to regulate normal cellular metabolism and enhance tolerance under abiotic stress (Ahmad, Ahanger, Alyemeni, Wijaya, & Alam, 2018). Furthermore, NO acts as a modulator of endogenous plant growth regulators/ phytohormones, and their synergistic or antagonistic effect directly or indirectly modulates the antioxidant system and helps to overcome stress (Ahmad et al., 2018). NO also reduce the toxicity of ROS through MAPK mediated phytohormones and establishing equilibrium between generation and detoxification of ROS by maintaining membrane stability and providing cell stability (Hasanuzzaman et al., 2017). Besides this, the level of NO (endogenous or exogenous application) has also been known for an essential factor in increased tolerance under abiotic stress (Ahmad et al., 2018). Being, involved in detoxifying ROS, some organelles are worked as localized accumulation of nitric oxide and reactive nitrogen species (RNS). At the cellular level, NO and H2O2 accumulation acts as indicator of stress and help in facilitating cellular adaptation to stress conditions.
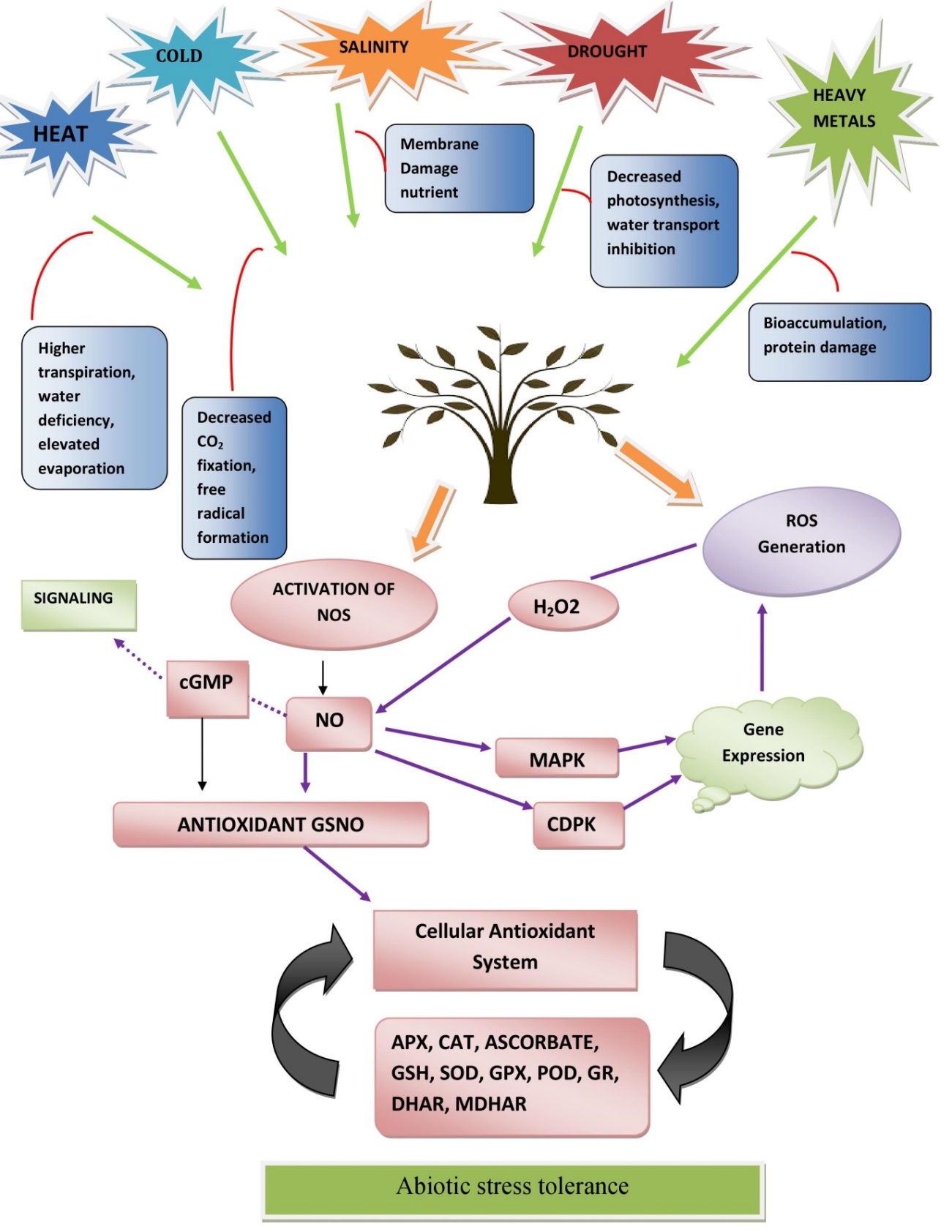
This organelle adaptation system removing over-burden under nitro-oxidative conditions, reducing cell harm and cell demise as well (Corpas & Palma, 2018). Plant peroxisomes have effective scavenging of ROS and RNS. These particles influence the usefulness of target proteins, peptides or unsaturated fats by means of post-translational changes (Corpas & Palma, 2018). In next context, we will discuss the ameliorative role of NO in stress condition and regulations of antioxidant enzyme and metabolic responses.
Nitric oxide as a Major Stress Impact-Mitigation Tool in Plants under abiotic stress
NO in Heavy metal (HM toxicity
Heavy metal toxicity is caused by copper, cadmium, aluminium, and arsenic, and it typically affects plant metabolic processes (Tiwari and Lata, 2018). The primary cause of metal accumulation in soil is the excessive use of agrochemicals, pesticides, fertilizers, irrigation of land with polluted water containing inorganic salts, and other natural or man-made activities (Masindi and Muedi, 2018). These metals enter plant cells via receptor molecules, contaminating important biomolecules such as DNA, RNA, and proteins, disrupting osmotic balances and reducing mineral nutrient requirements (Choudhury, Panda, Sahoo, & Panda, 2013). NO's regulatory mechanisms include osmotic balance by retaining cytoplasm organellar components, photosynthetic pigment retention, and photosynthetic efficiency, as well as enhanced tolerance to heavy metal toxicity (Ahmad et al., 2018). In RNA-seq-based transcriptomic analysis, fourteen heavy metal-related genes were found to have differential articulation in response to NO benefactor S-nitroso L Cysteine (CySNO) (Imran et al., 2016). Furthermore, the metal-nitrosylation-controlled cell-protective property of NO against metal-oxidative stress is a post-transitional modification (PTM). Table-1 is demonstrating the multifunctional role of NO in various abiotic stresses in different crops.
As previously discussed, endogenous NO levels increased under stress conditions as an adaptive approach. It was also reported that exogenous NO application induced the overexpression of stress genes in rice and mung bean under arsenic (As3+) contamination and mitigated the negative effect of metal toxicity by decreasing ROS and malondialdehyde (MDA) contents (Ismail, 2012). After treatment with arsenic, exogenous application of SNP to mung beans lowers the levels of lipid peroxidation, hydrogen peroxide, and maintains membrane integrity (Ismail, 2012). After treatment with arsenic, exogenous application of SNP to mung beans lowers the levels of lipid peroxidation, hydrogen peroxide, and maintains membrane integrity (Ismail, 2012). However, it is significant to note that the dose-dependent application of NO is a crucial parameter and demonstrated various responses. For instance, when applied under As (100 M) toxicity, SNP promotes -amylase activity in comparison to untreated SNP, which lowers the carbohydrate contents (Ismail et al., 2016). On the other hand, the exogenous administration of SNP and As (10 M) shown excellent combinations in raising the antioxidant activity of cadmium-treated soybean cell suspension (Kumar et al., 2017).
Additionally, aluminium toxicity (Al) reduces the uptake of minerals and nutrients, which reduces agricultural yield and productivity in acidic soils. The use of SNP has been demonstrated to reduce al toxicity in a dose-dependent manner via improve root development in Hibiscus moscheutos. Similar outcome has also been known where Cd-toxicity causes ROS production alters redox equilibrium and damages vital cellular components and macromolecules (Rasafi et al., 2022). Furthermore, Cd affects mineral and hormonal balances such as potassium and cytokinin levels, as well as osmotic imbalances and various metabolic pathways (Khanna et al., 2022). Furthermore, Cd toxicity had a lethal effect on roots and the normal functioning of mineral and nutrient uptake (Hédiji et al., 2015). SNP has a mitigating effect on ROS levels by improving antioxidant enzymes in Lolium perenne seedlings exposed to Cd toxicity (Arasimowicz-Jelonek, Floryszak-Wieczorek, & Gwóźdź, 2011). The collaborative studies proven that SNP inhibits the translocation of toxic metals inside the root of Arachis hypogeae, thereby increasing photosynthetic efficiency (Dong, Jinc, Liu, Xu, & Kong, 2014).
Plant |
Stress |
Application of NO |
Responses |
|
Fescue leaves |
Arsenic toxicity |
NO |
Elevated endogenous NO |
Jin et al., 2010 |
Pogonatherum crinitum |
Lead toxicity |
NO |
Enhance NR activity |
Yu et al., 2012 |
Triticum aestivum |
Heat stress |
NO |
Upregulating antioxidant defense system & methyl glyoxal (MG) detoxification system |
Hasanuzzaman et al., 2012 |
Zea mays |
Boron toxicity |
NO |
Enhanced antioxidant capacity |
Esim et al., 2013 |
Triticum aestivum |
UV-B radiation |
NO |
Enhanced ATPase activity and improve chlorophyll content |
Yang et.al., 2013 |
Triticum aestivum |
Salt stress |
NO |
Improved plant growth and antioxidant system |
Kausar et al., 2013 |
Tomato |
Salt stress |
NO |
Reduce lipid Peroxidation in leaves, increase the activity of antioxidant enzymes |
Manai et al., 2014 |
Sunflower plants |
Drought |
NO |
Increase the level of antioxidant compounds |
Cechin et al., 2015 |
Tomato seedling |
Chilling stress |
NO & polyamines |
Enhance NOS And NR activities indirectly induce H2O2 |
Diao et al., 2016 |
Chickpea |
Salt stress |
NO |
Induce stress-related Gene, enhance leaf related water content(LRWC) |
Ahmed et al., 2016 |
Mustard |
Salt stress |
NO &Sulphur |
Increase activity of ATP SULFARASE,CAT,APX,GR |
Fatima et al., 2016 |
Cowpea |
Lead toxicity |
NO |
Increase activity of antioxidant enzyme and increase proline accumulation |
Sadeghipour ,2016 |
Rice |
Arsenite phytotoxicity |
NO & SA |
Enhance Level Of Exogenous No (By 33% And 77%) |
Singh et al., 2017 |
Tomato |
Heat stress |
NO &Ca |
Enhance activities of RuBisco, antioxidant enzymes and stimulate biosynthesis of compatible solutes |
Siddiqui et al., 2017 |
Purslane |
Salinity& drought |
NO |
Reduce negative impact of stress |
Ekinchi et al., 2018 |
Canola plants |
Salinity stress |
SNP |
Mitigate injuries on plant growth and improve the biochemical process |
Farouk et al., 2018 |
Hyoscyamus niger |
Salt stress |
NO |
Increase antioxidant capacity |
Samsampour et al., 2018 |
Perennial ocye |
Cd toxicity |
NO |
Enhanced antioxidant system capacity |
Chen et al., 2018 |
NO in salinity stress
Salinity restricts plant growth and development and it was estimated that and salinity stress affects approximately 45 percent of arable land (Shrivastava & Kumar, 2015). Higher salt concentrations inhibit water uptake, while salt accumulation in the cytosol alters osmotic balances, causes ROS overproduction, halts metabolic processes (Fatma, Masood, Per, & Khan, 2016). NO-mediated stress mitigation has been extensively studied. For example, endogenous NO concentrations in Nicotiana tabacum plants have been shown to increase in response to salinity stress by modulation antioxidant and redox defense system in compliance with H2S (Silva, Fontes, Modolo, & V, 2017). The improved parameters includes maintenance of leaf relative water content, osmolyte regulation, enhanced photosynthetic pigments, and antioxidant defence system in Cicer aritinum (Ahmad et al., 2016). Furthermore, exogenous NO improved salt resistance by reducing oxidative stress, enhancing proton-pump and Na+/H+ antiport action in the tonoplast, and increasing the K+/Na+ proportion (Santisree, Bhatnagar-Mathur, & Sharma, 2015). NO influences saltiness resistance by controlling plasma membrane H+-ATPase and Na+/K+ proportion, resulting in an H+ slope that allows for Na+/H+ trade (Zhang et al., 2006). NO, for example, has been linked to increased K+, Ca2+, and Mg2+ content in salt-treated Gossypium hirsutum plants (Dong et al., 2014). The utilization of Diethylenetriamine NONOate (DETA/NO) enhanced long haul saltiness impacts in Glycine max through the enlistment of antioxidant agent chemicals (Egbichi, Keyster, & Ludidi, 2014). Studies have given evidence that NO interceded detoxification is incompletely by its capacity to direct ascorbate glutathione go through S-nitrosylation (Camejo et al., 2013). It is apparent from the examinations that salt pressure initiates an expansion in all-out S-nitrosylation particularly S-nitrosylation of the glycine dehydrogenase P subunit, F1 ATPase β subunit, and isocitrate dehydrogenase (ICDH) signifying the role of NO-intervened posttranslational alterations in controlling respiratory/photorespiratory pathways (Camejo et al., 2013; Fares, Rossignol, & Peltier, 2011). Then again, salt pressure-actuated O2 may the sameas decrease the S-nitrosylation level by interfacing with S-nitrosoglutathione (GSNO/RSNO) (Fancy, Bahlmann, & Loake, 2017). Also, the improved cell reinforcement compound movement due to a foliar spray of NO in different harvest plants has additionally brought about expanded plant development under salinity (Fatma & Khan, 2014). A marked decline was also reported in membrane permeability, intercellular CO2 concentration (Ci), malondialdehyde (MDA) and H2O2 by enhancing the activity of antioxidant enzymes, under salt stress. An enhancement in proline content was also observed under salinity (Lopez-Carrion, Castellano, Rosales, Ruiz, & Romero, 2008). Reports indicate that SNP application proves to be very useful in maintaining carbon and nitrogen metabolism by increasing total soluble protein through the enhancement of endopeptidase and carboxypeptidase activity, under salinity (Kong et al., 2016). In the roots of Phaseolus vulgaris, glucose-6-phosphate dehydrogenase is involved in NR-mediated NO biosynthesis under salt stress.
Furthermore, it was also reported that NO increased level of photosynthetic pigments, improved photosynthetic efficiency, and enhancing energy generation process in mitochondria under salinity (Ruan, Shen, Ye, & Xu, 2002). This was additionally discovered by improved photosynthesis by extinguishing overabundance vitality and expanding in quantum yield of PSII by utilizing exogenous NO in Solanum melongena seedlings (Wu et al., 2013). The exogenous use of NO has been accounted for to control the movement of phosphoenolpyruvate carboxylase kinase in Sorghum bicolor under salt stress (Monreal et al., 2013). NO application improved the photosynthetic capability of Brassica juncea under salt pressure (Fatma & Khan, 2014). The enhanced photosynthesis after NO application under salinity in Lycopersicon esculentum has been attributed to improved gas exchange parameters and chlorophyll fluorescence (Wu et al., 2013). It has also been well documented that NO and other phytohormones synergistically increased concentration of osmoprotectant molecules, soluble sugars, salt stress protein, thus confirming its role in mitigating the adverse effects of salinity stress (Ahmad et al., 2018).
NO in Drought stress
Drought or water scarcity has also been considered as the most significant stress that alters numerous physiological, morphological, biochemical processes of the developmental process (Wahab et al., 2022). Enumerate studies showed the production and accretion of NO in plants dependent upon the intensity and spite of drought (Santisree et al., 2015).The increased concentration of NO emerged as a vital element in drought resilience through improving the cell reinforcement frameworks, proline level, ROS, and osmolytes metabolisms (Shi, Ye, Zhu, & Chan, 2014). NO accumulation under water scarcity directly linked with decreasing in the frequency of stomata appearances and its closure to avoid water loss in Vitisv inifera (Patakas, Zotos, & Beis, 2010). Interestingly, it also stated that exogenous application of NO as donor stimulates the synthesis ABA (Zang et al., 2011). The stomata closure is also initiated through ABAstress hormone coupled with NO by activating signalling pathway via mitogen-activated protein kinase (MAPK), cyclic guanosine monophosphate (cGMP) and Ca2+messenger at molecular level (Shakihama et al., 2003; Gayatri et al., 2013). In response to drought, the plant exhibits DNA methylation and causes genome-wide alteration. The cellular and molecular changes such as overexpression of NO-responsive drought-related genes and antioxidant defense system and involvement of transcription factor upon exogenous application of SNP (Parani et al., 2004; Fan et al. (2012)Fan et al. (2012). Exogenously, NO promotes (ABA) by up-regulating specific gene expression (9-cis-epoxycarotenoid dioxygenase) and also showed feedback-inhibition on ABA affectability thus improving plant growth and showed enhanced resistance to drought pressure (Santisree et al., 2015). NO enhances drought tolerance when applied exogenously where it is postulated that NO donor enhances ABA synthesis (Zang et al., 2011).
Temperature Fluctuations
Sudden and severe temperatures alteration (heat, cold and chilling stress) causes irreversible cessation to seed germination, growth and metabolism, delayed flowering, and altered gene expression (Awasthi et al., 2016; Santisree et al., 2017). Excessive heat stressare responsible for enzyme inactivation, causes membrane damage, and initiate breakdown of biomolecules due to uncontrolled production of ROS and other superoxide ions (Suzuki & Mittler, 2006). The prominent role of NO to counter heat stress has been attained by S-nitrosothiols accumulation and enhanced activity enhancing NOS and GSNOR activity (Yu et al., 2014). For example, during heat stress combined action of NO, SNP and S-nitroso-N-acetylpenicillamine upgrade the antioxidant system and retains the loss caused by oxidative damage in leaves of Phragmitesaustralis (Song et al., 2016). The significance of NO homeostasis in heat stress resistance has been featured by a gene mutation in an atgsnor1-3/hot5-2 locus or RNAi line of Arabidopsis thaliana, where over a collection of NO corresponded with high warmth affectability (Parankusam, Bhatnagar-Mathur, & Sharma, 2017). Further, NO scrounger could ready to save the heat affectability of these mutant lines of Arabidopsis thaliana (Parankusam et al., 2017). Nonetheless, overabundance NO has been appeared to hinder electron transport by reversibly official to thylakoid layer buildings of Pisum sativum (Ziogas et al., 2013). Moreover, heat-instigated fundamental and effective changes in the thylakoid film regularly bring about ROS development (Pospı'ˇsil, 2016). A few investigations clear the capacity of NO in keeping up the cell redox homeostasis by killing hurtful ROS delivered by heat pressure (Ziogas et al., 2013). Be that as it may, delayed warmth stress was found to initiate nitrosative worry in Pisum sativum because of expanded S-nitrosylation (SNO) content (Parankusam et al., 2017). SNP pre-treatment additionally helped in osmotic alteration under warmth worry by up regulating the P5CS quality in Oryza sativa seedlings and decreasing putricine (PUT)/polyamine (PAs) proportion in Gingiber officinale (Uchida, Jagendorf, Hibino, Takabe, & Takabe, 2002). It was demonstrated that about 13 tyrosine-nitrated proteins including compounds like ferredoxin NADP oxidoreductase and carbonic anhydrase got incited by heat worry in Chrysanthemum morifolium seedlings (Chaki et al., 2011). Be that as it may, point by point worldwide atomic profiling by omics approaches is expected to expand our insight on the impact of NO and NO-interceded PTMs at the subcellular level to alleviate nitrosative impacts triggered by heat.
Chilling stress also alters biochemical and physiological processes as well as ROS-homeostasis in plants (Xin et al., 2008). Exogenously applied SNP ameliorates the decline in net photosynthetic rate and chlorophyll content, under freezing conditions (Tan et al., 2008). Not limited to this, SNP treatment reduced chilling damage in Cynodondactylon by diminishing the malondialdehyde (MDA) component and electrolyte emission because of the incitement of superoxide dismutase (SOD) and peroxidase (POD) responses (Fan et al., 2015). Additionally, exogenously applied SNP to cold focused on Triticum aestivum seedlings extended the resistance by upgrading the balance of cell reinforcement catalysts (Esim & Atici, 2014).
Interaction of NO with phytohormones to enhance abiotic tolerance
Plant growth and development is well-coordinated with hormonal regulation either synergistically or antagonistically under optimal or stress conditions (Ahmad et al., 2019). The interaction of NO with other growth hormones showed that NO in normal functioning of morphological and physiological process of plants (Sami et al., 2017). Involvements of NO are not restricted to interaction with PGRs, but also control its synthesis, regulation and transportation depending upon the internal and external conditions.In last decade, NO are regarded as most important messenger molecules in in the signalling pathways of developmental process. For instance, NO has been directly linked with salicylic acid, jasmonic acid, ethylene, auxins and cytokine during stomatal movement under ecological responses (Ahmad et al., 2020; Fatma & Khan, 2014). The interrelation of NO-ABA and Ca2+ in flowering and stomatal closing has already been discussed in earlier section. Crosstalk of NO and other plant growth regulator showed differential response under extreme ecological condition. In this context, positive correlation has been reported between NO and SA under salinity stress by preventing membrane leakage, reduced accumulations of malondialdehyde, 4-hydroxy-2-nonenal and protein carbonyl and increased accumulation of osmoprortectant (Yadu et al., 2017). The exogenous applications of NO enhance the biosynthesis of SA and consequently SA increased the production of H2O2 and NO (Alavi et al., 2014). Also, synergistic use of NO and SA improved Ca2+ /Mg2+ retention, elevated proline level while at the same time moderating the salt pressure foes in Glycine max seedlings.Correspondingly, the mix of NO and SA has been seemed to relieve the hurtful effects of Ni in Brassica napus and Cd in Arachis hypogaea (Xu, Fan, Dong, Kong, & Bai, 2015). It was represented that NO synthase is the genuine compound overwhelmingly remember for NO-generation. In Triticumaestivum leaves, NOA transcripts were up-managed by both ABA and NaCl treatment. It may be proposed that NOA1 protein is coordinated by ABA-related NO age (Hao, Xing, & Zhang, 2008). In Arabidopsis thaliana, similar crosstalk was seen between salicylic acid and NOA1-intervened NO age coordinated by ABA (Sun, Hao, Lu, & Ma, 2010). The stomatal conclusion is under direct control NO-reliant and NO-autonomous frameworks of ABA flagging system (Hancock et al., 2011). Additionally, high NO level is essential for stomatal conclusion yet at the same time higher NO focus prompts K+ efflux from the gaurd cells (Sokolovski & Blatt, 2004). In Zea mays leaves, ABA-actuated H2O2 production is required for a raised degree of NO subsequent in the institution of mitogen-started protein kinase and up-rule of cell fortification development (Mur, Prats, Pierre, Hall, & Hebelstrup, 2013).
Further, a positive interrelation among NO and auxins has been demonstrated in powerful in improving the ferric-chelate reductase development in a Fe-lacking Arabidopsis thaliana (Chen et al., 2010). Like auxin, a positive relationship among NO and CK under dry condition was represented, wherein the treatment with CK oversaw photosynthetic mechanical assembly by propelling NO motioning in Zea mays (Shao et al., 2010). Then again, CK diminished NO measurements to trigger stomatal opening in dim or dark condition Viciafaba seedlings (Santisree et al., 2015). Foliar utilization of SNP delayed salt-actuated leaf senescence by up-dealing with the proclamation of isopentenyltransferase (IPT) in Gossypiumhirsutum seedlings (Kong et al., 2016). Be that as it may, more examinations are required to give solid proof to NO and CK association under abiotic stress. Past examinations have point by point the participation of NO in SA-actuated stomatal end in Arabidopsis thaliana (Sun et al., 2010).
A negative association was seen between endogenous NO and ethylene during aging Perseaamericana and Fragaria (Simontacchi, Garcia-Mata, Bartoli, Santa-Maria, & Lamattina, 2013). The essential concordance among NO and ethylene is apparently basic to turn away cold-started harm during postharvest natural product aging and seed protection (Bai et al., 2011). Like SA, ET incited by Cd stress diminished NO levels in Pisum sativum and Glycine max seedlings. Moreover, both NO and ET are required to upregulate the layer H1-ATPase and another elective pathway to control ionic adjusts for improved salt flexibility (Wang et al., 2010). Additionally, NO likewise assumes a basic fundamental job in cell death programming and the hyponastic responses by starting ethylene biosynthesis during flooding pressure (Pasqualini, Reale, Calderini, Pagiotti, & Ederli, 2012). It is outstanding from the writing that sorted out action among NO and plant hormones including ABA, JA, GA, and CK, prompts thermo-versatility by improving the cell fortification framework and up-controlling the statement of warmth stun protein (Zandalinas et al., 2016). Further, pre-treatment of Zea mays seedlings with H2O2 immediately started endogenous H2O2, NO, and H2S assortment under warmth stress, which was exchanged by H2O2 forager dimethyl thiourea and NO scrounger cPTIO, indicating that H2O2 incited heat pressure strength was related with the crosstalk between downstream sections NO and H2S (Li et al., 2013b). ). A connection among NO and GA has been set up in advancing apical root development on Triticumaestivum under Al toxicity (He, He, Gu, & Li, 2012). Despite this evidence, the detail understanding of key vital engaged with NO atoms with other flagging particles requires further assessment to explain how NO correspondingly teams up with hormone-related proteins at the posttranscriptional or translational measurement.
Limitation and Future Prospective
NO has been regarded as a furtive molecule due to its active involvement in plant growth and development under both normal and stress conditions. In other words, applying NO exogenously from germination to maturity improves all physiochemical processes while mitigating the negative effects of abiotic stress. However, action and efficiency are dependent on concentration, endogenous level of NO, as well as metabolic process and plant developmental stage. Despite extensive research on NO's multifaceted role in plants, some dose-dependent action is known, such as how a concentration acts differently under different stress conditions, and the detailed mechanism is unknown. Another unanswered question is how the pressure explicit NO amassing converts into an organic capacity that aids in stress reduction. Furthermore, the interaction of NO with other plant growth regulators has been linked to the regulation of normal functioning in plant growth and development, such as mineral uptake and root development. Furthermore, the high throughput - omics investigation of NO-intervened pressure reactions is largely unexplored. More scientific research into recognisable NO involvement will illuminate the path taken by NO donors under stress conditions. Together, research into NO digestion and its communicating partners in plants, as well as their physiological significance under pressure, will help us gain a better understanding of NO capacities.
Data availability The datasets generated and/or analysed during the current study are not publicly available due but are available from the corresponding author on reasonable request.
Declarations
Ethics approval and consent to participate Not applicable.
Consent for publication Not applicable
Conflict of interests The authors declare that they have no known competing financial interests or personal relationships that could have appeared to influence the work reported in this paper.